Learn more: PMC Disclaimer | PMC Copyright Notice
The Emerging Role of the Sympathetic Nervous System in Skeletal Muscle Motor Innerfvation and Sarcopenia
Abstract
Examining neural etiologic factors’role in the decline of neuromuscular function with aging is essential to our understanding of the mechanisms underlying sarcopenia, the age-dependent decline in muscle mass, force and power. Innervation of the skeletal muscle by both motor and sympathetic axons has been established, igniting interest in determining how the sympathetic nervous system (SNS) affect skeletal muscle composition and function throughout the lifetime. Selective expression of the heart and neural crest derivative 2 gene in peripheral SNs increases muscle mass and force regulating skeletal muscle sympathetic and motor innervation; improving acetylcholine receptor stability and NMJ transmission; preventing inflammation and myofibrillar protein degradation; increasing autophagy; and probably enhancing protein synthesis. Elucidating the role of central SNs will help to define the coordinated response of the visceral and neuromuscular system to physiological and pathological challenges across ages.
This review discusses the following questions: (1) Does the SNS regulate skeletal muscle motor innervation? (2) Does the SNS regulate presynaptic and postsynaptic neuromuscular junction (NMJ) structure and function? (3) Does sympathetic neuron (SN) regulation of NMJ transmission decline with aging? (4) Does maintenance of SNs attenuate aging sarcopenia? and (5) Do central SN group relays influence sympathetic and motor muscle innervation?
Introduction
Age-dependent decline in muscle mass, force and power, termed sarcopenia,(Rosenberg, 1989) impairs mobility to increasing the risk of falls, institutionalization, comorbidity, and premature death.(Larsson et al., 2019; Tieland et al., 2018) In addition to impairing quality of life in the adult population, sarcopenia is also a major risk factor for many age-associated diseases.(Chin et al., 2013; DeAndrade et al., 2018; Landi et al., 2012; Maeda and Akagi, 2016; Nishiguchi et al., 2016; Srikanthan et al., 2010; Tournadre et al., 2019; Upadhya et al., 2015) Sedentary people lose 0.5 percent of lean muscle mass every year between age 25 and 60; the rate doubles to about 1 percent after 60, and doubles again each decade thereafter.(Lynch et al., 1999) Once muscle mass in older adults falls below two standard deviations of the mean of young controls and gait speed falls below 0.8 m/s, a clinical diagnosis of sarcopenia is reached.(Studenski et al., 2011) Although, the average population undergoing sarcopenia varies according to the nutritional status and physical activity, more than 50% of people over 80 undergo sarcopenia,(von Haehling et al., 2010) and all others exhibit some degree of muscle atrophy and loss of muscle function.(Larsson et al., 2019) Those with sarcopenia subjects are not necessarily frail; sarcopenia is about twice as common as frailty.(von Haehling et al., 2010)
Physiological and pathological studies show that innervation and systemic factors exert a strong influence on skeletal muscle mass, composition and function.(Delbono, 2003; Larsson et al., 2019) Sarcopenia is associated with: (a) excitation-contraction uncoupling,(Delbono, 2011a; Delbono et al., 1995; Wang et al., 2002) which diminishes muscle specific force (force normalized to cross-sectional area); (Gonzalez et al., 2000a; Gonzalez et al., 2000b; Wang et al., 2000) (b) decreased number of muscle fibers as well as fiber atrophy; (Dutta, 1997; Lexell, 1995) (c) changes in fiber type; (Frontera et al., 2000; Larsson et al., 1991) (d) diminished muscle power related to decreased maximal isometric force and slower sliding of actin on myosin; (Brooks and Faulkner, 1994; Hook et al., 1999) (e) impaired recovery after eccentric contraction; (Faulkner et al., 1993) and (f) deposit of intra- and extra-myocellular lipids with consequent changes in skeletal muscle quality.(Choi et al., 2012) Motor and/or sympathetic muscle denervation trigger and/or exacerbate some of these processes.
Explanations for these age-dependent changes in muscle properties include: alterations in myofiber calcium homeostasis,(Delbono, 2011b; Jimenez-Moreno et al., 2008; Tang et al., 2011) decreased muscle loading associated with sedentary lifestyle, (Landi et al., 2014),(Tseng et al., 1995) oxidative damage, (Jackson, 2016; Jang et al., 2010; Leeuwenburgh et al., 1998; Vasilaki et al., 2017; Weindruch, 1995) impaired mitochondrial quality control,(Picca et al., 2019) age-dependent decrease in insulin-like growth factor-1 (IGF-1) expression or tissue sensitivity, (Owino et al., 2001; Renganathan et al., 1997; Schiaffino et al., 2013) hormonal and metabolic alterations,(Kamwa et al., 2020; Vitale et al., 2016) impaired autophagy,(Masiero et al., 2009; Sakuma et al., 2016; White et al., 2016; Wokke et al., 1990) accumulation of senescence cells,(Tchkonia et al., 2013; Zhu et al., 2014) inflammation,(Ferrucci et al., 1999; Ferrucci et al., 2002; Tchkonia et al., 2013) decline in satellite cell proliferation, (Garcia-Prat et al., 2016; Hwang and Brack, 2018) and loss of neural control in skeletal muscle.(Delbono, 2003) Unfortunately, some of these mechanisms are technically difficult to test in the whole organism, particularly in mammalian species. Pharmacological interventions produce numerous side effects, have yielded inconsistent results, and their rationales have been challenged.(Fry et al., 2015; Jackson, 2016; Larsson et al., 2019; Ristow, 2014; Sakellariou et al., 2017; Wang and Hekimi, 2015) Still, the interaction between nerve and skeletal muscle is clearly crucial for both tissues to survive and function adequately throughout life.(Delbono, 2003; Payne et al., 2006b) Does muscle atrophy and weakness result from primary neural or muscular etiologic factors or a combination? Does age-dependent maintenance of sympathetic neuron transcription factors, particularly the heart and neural crest derivative 2 (Hand2), (Hendershot et al., 2008; Rodrigues et al., 2020; Stanzel et al., 2016) attenuate motor denervation? Examining neural etiologic factors’ role in the decline of neuromuscular function with aging is essential to our understanding of the mechanisms underlying sarcopenia.
This review focuses on how aging influences skeletal muscle sympathetic and motor innervation and how their alteration mediates sarcopenia pathogenesis. It discusses basic aspects of muscle innervation, even at a young age, that are incompletely understood. This review is organized to consider the following questions: (1) Does the sympathetic nervous system (SNS) regulate skeletal muscle motor innervation? (2) Does the SNS regulate presynaptic and postsynaptic neuromuscular junction (NMJ) structure and function? (3) Does sympathetic neuron regulation of NMJ transmission decline with aging? (4) Does maintenance of sympathetic neurons attenuate aging sarcopenia? and (5) Do central sympathetic neuron group relays influence sympathetic and motor muscle innervation?
(1) Does the SNS regulate skeletal muscle motor innervation? Relationship between sarcopenia and the dual muscle innervation
Sarcopenia is characterized by heterogeneous muscle fiber size and myosin heavy-chain composition,(Schiaffino, 2018; Schiaffino and Reggiani, 1994) selective myofiber atrophy, myofiber grouping, and progressive NMJ degeneration, leading to complete or partial synapse denervation, and Schwann cell depletion.(Delbono, 2003; Li and Thompson, 2011; Wang et al., 2005) Despite its relevance to the onset and outcome of sarcopenia, the etiology of age-related skeletal muscle denervation remains unknown.(Delbono, 2011a) Several groups have reported skeletal muscle denervation and reinnervation and motor unit remodeling or loss in aging rodents or humans. (Doherty et al., 1993; Einsiedel and Luff, 1992; Hashizume et al., 1988; Johnson et al., 1995; Kanda and Hashizume, 1989, 1992; Larsson and Ansved, 1995; Larsson et al., 1991; Larsson et al., 2019; Larsson and Edstrom, 1986; Zhang et al., 1996) Motor-unit remodeling leads to changes in fiber-type composition. (Pette and Staron, 2001; Schiaffino and Reggiani, 1994, 1996)
During development, muscle fiber phenotype is determined by interactions among subpopulations of ventral spinal cord motor neurons that activate contraction at rates ranging from 10 (slow fibers) to 100 (fast-fatigue resistant) to 150 Hz (fast-fatigue sensitive).(Buller, 1960a, b; Greensmith and Vrbova, 1996) During aging, motor-unit remodeling appears to involve denervation of fast muscle fibers and their reinnervation by axonal sprouting from slow fibers.(Frey et al., 2000; Kadhiresan et al., 1996; Larsson, 1995; Lexell, 1995) When denervation exceeds reinnervation, that population of muscle fibers becomes atrophic and non-functional. Although denervation contributes to skeletal muscle atrophy and functional impairment with aging,(Larsson and Ansved, 1995) its time course, and prevalence in human and animal models of aging remain undetermined. Combining contraction force recordings with muscle immunostaining for the neural cell-adhesion molecule (NCAM) protein, a marker of fiber denervation and reinnervation in rat studies showed that the old have significantly more denervated fibers than the young. (Andersson et al., 1993; Gosztonyi et al., 2001) Moreover, the area of denervated fibers, detected by positive staining with NCAM antibodies, accounts for a significant fraction of the decline in specific force. (Urbancheck et al., 2001) Human skeletal muscle studies confirm NCAM’s usefulness as a denervation/ reinnervation marker. (Biral et al., 2008; Messi et al., 2016; Sonjak et al., 2019)
We reported that muscle denervation in aging mice is more extensive than previous functional and structural assays predicted. Two sodium channel isoforms are expressed in skeletal muscle, the TTX-sensitive Nav1.4 or SkM1 and the TTX-resistant Nav1.5 or SkM2.(Kallen et al., 1990; Trimmer et al., 1989) The SkM2 mRNA level is highest in early development, when TTX-insensitive channels predominate, but declines rapidly with age as SkM1 mRNA increases; SkM2 mRNA is not detectable in normally innervated adult skeletal muscle but increases greater than 100-fold after denervation; rat cardiac muscle has abundant SkM2 mRNA but no detectable SkM1 message.(Kallen et al., 1990) To assess the extent of denervation in aging rodents, we combined electrophysiological and immunohistochemical assays to detect the expression of TTX-resistant Nav1.5 in flexor digitorum brevis muscles from young-adult and senescent mice. Sodium current density measured with the macropatch cell-attached technique did not show significant differences between muscle fibers from young and old mice. The TTX dose-response curve, using the whole-cell voltage-clamp technique, showed three populations of fibers in senescent mice: one similar to fibers from young mice (TTX sensitive); another similar to fibers from experimentally denervated muscle (TTX resistant); and an intermediate group. Partially and fully denervated fibers made up approximately 50 percent of the total tested, which aligns with the percentage of fibers positive for the Nav1.5 channel, as shown by specific immunostaining.(Wang et al., 2005) Discrepant results related to the time course, location, and extent of denervation in rodents (Hendrickse et al., 2018) can be accounted for by the age of the animals and the denervation and immunofluorescence techniques used. These studies revealed that new technical approaches are needed to accurately assess muscle denervation, which, although subtle, can be devastating over time. (Delbono, 2003)
Increased frequency of hybrid fiber types and quantification of myosin heavy chain (MHC) composition in aging skeletal muscle
Since innervation regulates MHC expression,(Schiaffino and Reggiani, 1996) muscle morphometric analysis of MHC predominance is critical to understanding the myofiber/motoneuron relationship in health and disease.(Lefeuvre et al., 1996) Determining fibers’ shapes, sizes, and arrangements elucidates muscle tissue morphology and overall health,(Gropp, 2017) while immunohistochemistry (IHC) using MHC antibodies is a widely accepted approach to examine skeletal muscle fiber type composition.(Bloemberg and Quadrilatero, 2012) However useful for compositional studies of larger portions of the body, computed tomography (CT) scan, magnetic resonance imaging (MRI), and dual-energy X-ray absorptiometry cannot visualize the microscopic structural features of skeletal muscle. Performed with the skill needed to correctly interpret and generate reproducible results, IHC offers the best combination of molecular and microscopic morphological information for the analysis of skeletal muscle.
Both semiautomatic and automatic approaches have been developed to aid IHC analysis;(Bergmeister et al., 2016; Briguet et al., 2004; Lau et al., 2018; Mayeuf-Louchart et al., 2018; Miazaki et al., 2015; Pertl et al., 2013; Smith and Barton, 2014; Wen et al., 2018) but aging skeletal muscle poses difficulties that young, healthy muscle tissue does not. Failing autophagy leads to intracellular protein deposits.(Jokl and Blanco, 2016) Aging skeletal muscle usually displays some degree of atrophy; more fibers express more than one MHC (hybrid); interstices are infiltrated with fat cells, lipofuscin, and fibrous tissue, and angulated small myofibers disperse heterogeneously throughout the tissue.(Distefano and Goodpaster, 2018; Hepple, 2018; Purves-Smith et al., 2014) These characteristics can affect the efficacy of muscle analysis programs that rely on tissue homogeneity.
We designed a reliable, user-friendly, and accessible approach to quantifying basic muscle morphometric parameters that does not require computer experts or statisticians.(Bonilla et al., 2020) It emphasizes image thresholding and editing capability to properly quantify hybrid fibers and to identify muscle fibers, regardless of their degree of atrophy, respectively.(Bonilla et al., 2020)
Plasticity at the aging neuromuscular junction
NMJ formation involves a number of resident molecules, such as low-density lipoprotein (LDL)- related receptor protein 4 (LRP4),(Messéant et al., 2017; Weatherbee et al., 2006) agrin (differentiation), (Ruegg and Bixby, 1998) Dok-7 (synaptogenesis),(Okada et al., 2006) and muscle-specific kinase (MuSK) (synapse maintenance),(Koneczny et al., 2014) and others. MuSK participates in three signaling pathways: Agrin-LRP4, Wnt, and MuSK-BMP, via distinct domains. Although genetic studies have elucidated the role these proteins play in NMJ structure and function, how they contribute to its age-associated functional decline remains ill-defined.(Fish and Fallon, 2020) Age-associated structural and functional alterations of the neuromuscular junction such as instability of neuromuscular junction have been reported.(Balice-Gordon, 1997; Khan et al., 2016; Payne and Delbono, 2004; Payne et al., 2006a; Rodrigues et al., 2018; Valdez et al., 2010) The events leading to denervation in aging less understood than the formation and activity‐ dependent editing of neuromuscular synaptic connections.(Personius and Balice-Gordon, 2000). Activity has been shown to play a crucial role, (Personius and Balice-Gordon, 2000) prompting the interesting hypothesis that a similar mechanism affects senescent mammals. While exercise and calorie restriction attenuate NMJ spatial distortion in aging mice and humans,(Messi et al., 2016; Valdez et al., 2010) the levels required are highly variable and difficult to sustain. Such interventions must be personalized and closely monitored.
Nerve terminal remodeling during the period of target absence does not adequately explain the subsequent changes to the NMJ. Mechanisms for remodeling the synapse include failure of the regenerating muscle fiber to contact the old basal lamina and nerve terminal, growth of the nerve terminal and its glia toward the regenerating fiber, and remodeling of the initial contact as the motor nerve terminal becomes varicose.(Li and Thompson, 2011)
In skeletal muscles from aging rodents, motor denervation causes reorientation of costameres (rib‐ like structures formed by dystrophin and β‐ dystroglycan),(Bezakova and Lomo, 2001) proliferation of triadic membranes,(Salvatori et al., 1988) reduction of voltage-gated calcium channels, and alterations to the sarcoplasmic reticulum Ca2+ release channel.(Delbono, 1992; Delbono et al., 1997; Delbono and Stefani, 1993; Wang et al., 2000) Costameric proteins transmit lateral forces and provide structural integrity in contracting mechanically loaded muscle fibers.(Straub and Campbell, 1997) Along with muscle activity, muscle agrin at a concentration two orders of magnitude lower than the effective concentration of neural agrin, regulates the organization of cytoskeletal proteins in skeletal muscle fibers.(Bezakova and Lomo, 2001) These molecular changes in aging muscle should be explored.(Taetzsch and Valdez, 2018) Compared to young and middle-aged mice, aging C57BL/6 mice show an increase in agrin, calcitonin gene-related peptide, growth associated protein 43, fibroblast growth factor binding protein 1, and transforming growth factor-β1at the NMJs of the tibialis anterior, soleus, extensor digitorum longus, and gracilis muscles as evidence of increased development, plasticity, and maintenance of NMJ signaling with aging. (Blasco et al., 2020)
The studies reported above strongly associate neural alterations with the onset and gradual decline of aging skeletal muscle function. Interventions focused on motor neurons, their axons, and associated non‐ neuronal cells, and the NMJ may slow or even reverse age‐ related impairments of skeletal muscle.
Dual innervation of the skeletal muscle
Unlike the skeletal motor system, which innervates striated muscles, the autonomic nervous system (ANS) innervates the smooth muscle organs and tissues.(Powley, 2008) Although skeletal muscle sympathetic muscle innervation was described over a century ago;(Boeke, 1909) myofiber sympathetic innervation remained unexplored, probably due to technical limitations. Sympathetic axons travel through the peripheral nervous system together with motor and sensory axons and then surrounding blood vessels.(Eichmann and Brunet, 2014; Wang et al., 2020a) to reach the muscle fiber.(Griffin and Thompson, 2008)
Sympathectomy and sympathetic neuron-labeling experiments using the monosynaptic retrograde tracer β-subunit of cholera toxin (CTβ) helped to elucidate the innervation of mouse hindlimb muscles by paravertebral sympathetic ganglia and ventral horn motoneurons. (Card and Enquist, 2012; Kerman et al., 2003; Rodrigues et al., 2018) For a long time, sympathetic innervation of skeletal muscle was thought to be restricted to the blood vessels until it was observed in the intra‐ and extrafusal fibers of several muscles. (Barker and Saito, 1981) This finding sparked researchers’ interest in the role of postganglionic sympathetic neurons and their neurotransmitter(s) in myofiber composition, trophism, and function. Even at birth, sympathetic innervation and interaction with NMJs in hindlimb, diaphragm, and levator auris longus muscles is extensive and, in the extensor digitorum longus muscle, increases until adulthood.(Straka et al., 2018)
The ANS coordinates homeostasis through sympathetic and parasympathetic outflows and the enteric nervous system.(Robertson, 2012) The SNS regulates the function of many tissues, and its genetic, autoimmune, or degenerative alteration results in a variety of clinical disorders affecting the brain, spinal cord, and/or nerve structure and function. While it also plays a role in skeletal muscle regeneration after injury(Beitzel et al., 2007) and the ability to perform short intensive tasks like the Wingate test,(Le Panse et al., 2007) its deterioration with age results in incapacitating symptoms.(Delbono, 2003; Gonzalez-Freire et al., 2014; Lipsitz and Novak, 2008) Its functional impairment and the resulting muscle weakness are manifestations of Alzheimer’s disease(Jensen-Daham et al., 2015) and such synucleinopathies as Parkinson’s disease, dementia with Lewy bodies, multiple system atrophy, and pure autonomic failure.(Askmark et al., 2001; Cano-Jaimez et al., 2010; Femminella et al., 2014; Janig, 2012; Kandinov et al., 2011; Low and Bennarroch, 2008; Mu et al., 2013; Munver and Volfson, 2006; Okamoto and Biaggioni, 2012; Ooi et al., 1997; Orimo et al., 2007; Tachi et al., 1995) Muscle weakness associated with autonomic dysfunction is also obvious in adrenal insufficiency, Lambert‐ Eaton myasthenic syndrome, and chronic fatigue syndrome.(Beitzel et al., 2007; Lindquist and Stangel, 2011; O’Suilleabhain et al., 1998) Aging produces several changes in the ANS that may impair adaptations to common physiologic stressors and increase the risk of developing diseases that further harm autonomic function.
We used a microsurgical approach to muscle sympathetic denervation to define the role of the SNS in regulating NMJ stability and function in adult mice.(Khan et al., 2016; Rodrigues et al., 2019) A decrease in synaptic vesicle release and quantum content indicates that motor axon function is impaired. Next, to determine how sympathetic and motor axons in the spinal nerve communicate under normal and pathological conditions, we examined whether sympathectomy alters myelination, myelin thickness, and/or neurofilament (NF) phosphorylation in large axons like those in motor neurons. We found increased variation in myelin thickness, probably a result of Schwann cell reprogramming.(Jessen and Mirsky, 2016) Myelinating Schwann cells modulate NF phosphorylation, axonal caliber, and slow axonal transport.(de Waegh et al., 1992) Although our analysis at the sciatic‐ tibio‐ peroneal nerve showed no downregulation of genes encoding axonal myelination, we found a reactive increase in neurotrophin gene transcription. NFs are abundant in motor and other neurons with large-diameter axons.(Ohara et al., 1993; Sakaguchi et al., 1993) The mechanism underlying crosstalk between sympathetic and motor axons remains unclear. The interaction of both axons with dephosphorylated NFs, revealed by transmission electron microscopy, may interfere with microtubules’ transport of enzymes and peptide precursors.(Purves et al., 2011)
The influence of the SNS on muscle motor innervation and acetylcholine receptor stability is extensive. It regulates NMJ transmission, maintains optimal Gαi2 expression, and prevents any increase in histone deacetylase 4 (Hdac4), myogenin, the muscle RING-finger protein-1 MuRF1 (a.k.a. TRIM63), and muscle-specific microRNA-206 (miR-206). The SNS also prevents elevations in MuRF1 levels, muscle atrophy, and maintains postsynaptic membrane acetylcholine receptor (AChR) levels, (Rodrigues et al., 2018) as depicted in Figure 1.
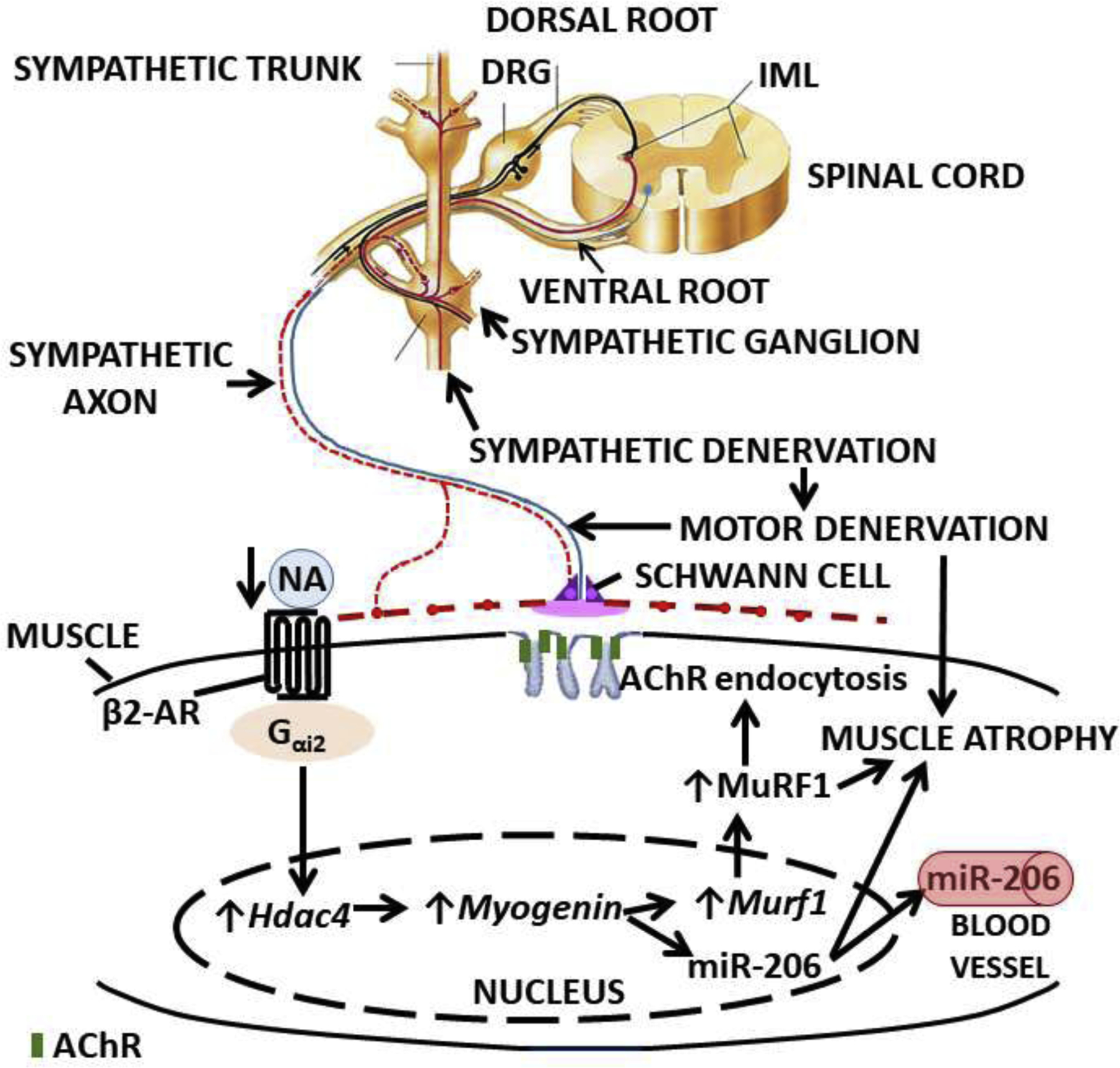
(Rodrigues et al., 2018) AChR: acetylcholine receptor; DRG: dorsal root ganglion; Hdac4: histone deacetylase-4; IML: intermediolateral column; NA: noradrenaline.
After sympathectomy, levels of the neurotransmitter noradrenaline (NA) decreased 80 percent or more in soleus and EDL muscles, while plasma catecholamines were not affected,(Silveira et al., 2014) which indicates that the surgical removal of lumbar paravertebral sympathetic ganglia did not prevent the adrenal gland medulla from releasing adrenaline into the bloodstream. Since the β2‐ AR (adrenergic receptor) is a heterotrimeric guanine nucleotide‐binding protein (G protein)‐ coupled receptor, and muscle NA levels and receptor activity decrease with sympathectomy,(Silveira et al., 2014) we examined whether sympathectomy led to decreased Gαi2 activation, a mechanism postulated to activate myogenin.(Mei et al., 2011) Minetti et al. reported that Gαi2 promotes skeletal muscle hypertrophy, myoblast differentiation, and muscle regeneration, indicating that receptors that act through Gα(i2) might be targets to prevent skeletal muscle wasting.(Minetti et al., 2014)
We found Gαi2 significantly decreased in hindlimb mouse muscles after sympathectomy, and that restoring it using a human Gαi2 (Q205L) mutant, subcloned into an adeno-associated viral vector, prevented decreases in membrane AChR levels and increases in MuRF1, Hdac4, myogenin, and β2‐ AR expression. These results indicate that increasing muscle levels of Gαi2 prevents skeletal muscle motor denervation.(Rodrigues et al., 2018)
The SNS regulates skeletal muscle motor innervation
To address whether the SNS regulates skeletal muscle motor innervation, we studied the impact of regional and systemic sympathetic ablation on muscle motor innervation using transcriptome analysis, retrograde tracing of the sympathetic outflow to the skeletal muscle, and electrophysiological, imaging, and protein analysis of NMJ transmission. We and others also used state-of-the-art microsurgical techniques in several wild-type and transgenic mice, including C57BL6, enzyme E3 ubiquitin-protein ligase TRIM63/muscle specific RING finger 1 (MuRF1KO), and dopamine beta-hydroxylase (DBH)-tomato and Thy-1 mice.(Khan et al., 2016; Rodrigues et al., 2019)
After SNS ablation, we found elevated levels of MuRF1 transcript and protein. MuRF1 is involved in muscle trophism and maintenance.(Moriscot et al., 2010) It is expressed in both type‐ I and type‐ II fibers, preferentially the latter; highly enriched in the postterminal; and interacts with AChR in endocytic structures.(Rudolf et al., 2013) The procedure evoked an increase in E3 ligase that led to an overall increase in AChR but a decrease in sarcolemmal AChR, indicating that sympathetic neurons influence AChR partition between the sarcolemmal and subsarcolemmal compartments. Moreover, sympathectomized MuRF1KO mice showed AChR partitioned much as it is in innervated young mice.(Rodrigues et al., 2018) These results support the conclusion that MuRF1 mediates SNS regulation of the fraction of receptors located at the postterminal NMJ sarcolemma.
Severing the sciatic nerve is considered a model of motor muscle denervation since flaccid paralysis and massive atrophy ensue.(Bodine et al., 2001; Carlson et al., 2002; Machado et al., 2019) Rapidly, following nerve transection, MuRF1 and F-Box Protein 32 (Fbxo32, a.k.a. atrogin‐ 1) increase,(Bodine et al., 2001) while at day 14, MuRF1, but not Fbxo32, was significantly increased after motor denervation in both soleus and tibialis anterior muscles as determined by immunoblot.(Moriscot et al., 2010) Note that treatment with the β2‐ AR agonist clenbuterol prevents denervation‐ induced increases in Fbxo32 levels.(Goncalves et al., 2012) These results indicate that maintaining sympathetic activity or stimulating β2‐ AR prevents Fbxo32 levels from rising and that muscle sympathetic ablation mimics, to some extent, muscle motor denervation and may account for changes in muscle gene expression previously attributed primarily to motoneuron ablation.
Whether MuRF1 mediates muscle atrophy is controversial. Transgenic muscle‐ specific overexpression of MuRF1 does not cause muscle atrophy, (Hirner et al., 2008) but the same group demonstrated that MuRF1 KO dramatically prevents denervation‐ induced atrophy in mouse TA muscle.(Moriscot et al., 2010) More recently, that induction of genes associated with the NMJ is blunted in MuRF1 KO mice has been reported.(Furlow et al., 2013) Thus, we cannot rule out MuRF1 participation in denervation‐ induced muscle atrophy.
The increase in MuRF1 after sympathectomy suggests that the SNS inhibits its expression under physiological conditions. We ruled out the participation of several alternative pathways,(Bonaldo and Sandri, 2013) and a potential effect of β2‐ AR.(Rodrigues et al., 2018) We also found an increase in protein kinase regulatory subunit-I alpha (PKA RIα) but a decrease in the PKA(RIIα)/PKA(RIα) ratio, which we recently proposed as a marker of the failure of postsynaptic AChR recycling and membrane insertion.(Xu et al., 2017) An alternative mechanism for the elevation in MuRF1 levels after SNS ablation could be a decrease in muscle autophagy. However, although lysosome degradation of proteasomes has been demonstrated in rat liver (Cuervo et al., 1995) its role in the skeletal muscle is not known.
The elevated Hdac4 concentration at the NMJ further supports the concept that sympathetic ablation impairs motor axon function.(Cohen et al., 2007; Moresi et al., 2010) MuRF1 regulation by the canonical Gαi2‐ myogenin cascade has been reported, (Minetti et al., 2014) but whether SNS dysfunction is involved is unknown. We found that sympathectomy downregulates Gαi2, which has been shown to increase Hdac4, (Minetti et al., 2014) and infecting the TA muscle with the constitutively activated form of Gαi2 (Moxham and Malbon, 1996) using an adeno‐ associated virus, AAV‐ Gαi2(Q205L), prevented both. Myogenin induces muscle and serum miR‐ 206, which suppresses Hdac4 and promotes reinnervation.(Moresi et al., 2010) SNS denervation of blood vessels may affect NMJ molecular composition and function, but specific Gαi2(Q205L) expression in myofibers (Minetti et al., 2014) is consistent with a direct sympathetic effect on NMJs. Also, muscle-flow recordings have shown that epinephrine’s effect on neuromuscular transmission is independent of concomitant vascular changes produced by the catecholamine.(Bowman and Raper, 1966)
(2) Does the SNS regulate presynaptic and postsynaptic NMJ structure and function? Sympathomimetics can be used to answer this question.
To explore sympathetic neuron-motoneuron cross-talk and its influence on NMJ transmission, we tested the effects of the sympathetic postganglionic neurotransmitter, noradrenaline, and sympathomimetics on such motoneuron-terminal targets as TRPV1 and P/Q- and N-type voltage-activated Ca2+ channels and the resulting Ca2+ fluxes and synaptic vesicle release dynamics. (Khuzakhmetova and Bukharaeva, 2020; Kuba, 1970; Rodrigues et al., 2018; Tsentsevitsky et al., 2019) The impact of SNS ablation and sympathomimetics on the amplitude of the compound muscle action potential has also been reported.(Khan et al., 2016)
Catecholamines act on specific receptors located on the cell surface of target organs. These receptors, expressed throughout the body, are classed in two groups. The α-subtype includes the Gq-coupled receptors α1A, α1B, and α1D and Gi-coupled receptors α2A, α2B, and α2C. The β-subtype comprises the Gs-coupled β1 and Gs/Gi-coupled β2 and β3 receptors. β1 and β2B are particularly important in understanding the mechanisms by which the SNS regulates NMJ transmission,(Rodrigues et al., 2019; Wang et al., 2020a) and β2 directly regulates skeletal muscle mass and metabolism.(Lynch and Ryall, 2008)
Noradrenaline (NA), the main neurotransmitter of ganglionic sympathetic neurons, modulates neuromuscular transmission.(Kuba, 1970) Regional surgical removal or systemic chemical ablation of mouse hindlimb SNS, causes a dramatic decrease in muscle NA,(Silveira et al., 2014) and the release of synaptic vesicles.(Rodrigues et al., 2018) Administration of sympathomimetic agents prevents the effects of chemical sympathectomy and reestablishes the compound muscle action potential disrupted by SNS ablation, demonstrating that SNS innervation controls NMJ homoeostasis.(Khan et al., 2016) Their use in vivo and ex vivo helped us to define the acute and chronic effects of the SNS on muscle function, and investigating how postganglionic sympathetic axons and their neurotransmitter(s) affect myofiber composition and function clarified the process of aging sarcopenia. β2-AR agonists increase protein synthesis in denervated muscle and after injury,(Goncalves et al., 2012) by suppressing proteolysis.(Navegantes et al., 2009) While the postsynaptic mechanism is well-established, these findings reveal a presynaptic mechanism based on crosstalk between the motor and sympathetic nervous systems at the NMJ.
The discovery prompted investigation of NMJ presynaptic vesicle release in response to acute ex-vivo or chronic, repetitive in-vivo administration of β-AR agonists. We focused on their effects on extracellular Ca2+ concentration, TRPV1, and P/Q- and N-type voltage-activated Ca2+ channels in young adult mice to determine how the SNS influences NMJ transmission.(Rodrigues et al., 2019)
If sympathomimetic agents enhance NMJ transmission and remediate muscle wasting and strength in aged rodents,(Carter et al., 1991; Ryall and Lynch, 2008) why do endogenously secreted catecholamines fail to maintain muscle mass and force in the absence of significant changes in β2-AR levels? Recent studies support a novel mechanism by which motor and sympathetic neurons interact at the NMJ presynapse.(Khan et al., 2016; Rodrigues et al., 2018; Rodrigues et al., 2019; Wang et al., 2020a) Impaired neuronal crosstalk can explain, at least partially, progressive atrophy and weakness with aging.(Rodrigues et al., 2019)
We examined whether the SNS regulates motor neuron axon function and neuromuscular transmission in situ—without any exogenous intervention, including delivery of catecholamines or sympathomimetic agents. Using endogenous neurotransmitters to regulate motoneuron synaptic vesicle release allowed fine-tuning of the acute stress response under physiological conditions. Our comprehensive approach included nerve immunolabeling co-registration and electrophysiological recordings in a novel mouse ex-vivo preparation that preserves the complexity of the interaction between motoneuron and SNS at the NMJ and muscle innervation in the living mouse. Defining the mechanisms by which the SNS regulates neuromuscular properties may have broad health implications, particularly if we can learn how to counteract sarcopenia, which impairs gait and mobility in older adults and people suffering from neurodegenerative diseases.(Wang et al., 2020a)
Figure 2 shows how sympathetic axon depolarization leads to N-type Ca2+ channel activation, Ca2+ influx, and NA release. This neurotransmitter then acts on sympathetic axon α2B- and β1-AR to inhibit N-type Ca2+ channels mainly through Gi and possibly exclusively via G(o)/G(i) and activate them through Gαs. N-type VGCC Ca2+ influx levels may result from the balance between yohimbine-sensitive α2-AR autoregulation and β1-AR activation. Other rapid pertussis toxin (PTX)-insensitive G-protein pathways may involve NA-independent activating mechanisms (G#).(Mirotznik et al., 2000) Propranolol, a β1/β2-AR blocker, and atenolol, a β1- AR blocker, prevent β1-AR activation and diminish NA release at the NMJ.(Wang et al., 2020b)

AP: axon potential. Red arrows indicate decreased function or protein expression with aging. Dashed lines indicate proposed pathways. Adapted from (Rodrigues et al., 2019; Wang et al., 2020a). AP: action potential; EPP: end-plate potential; NA: noradrenaline; QC: quantal content; tSC: terminal Schwann cell; VGCC: voltage-gated calcium channel.
Since NA has no effect on miniature end-plate potential (MEPP) frequency, end-plate potential (EPP) amplitude, and quantal content in the presence of propranolol,(Rodrigues et al., 2019) we proposed the motoneuron as a novel target of the SNS. We also proposed that Ca2+ influx through TRPV1 channels and P/Q-type Ca2+ channels (Cav2.1) determines the axoplasmic Ca2+ concentration at rest and during motor neuron depolarization, respectively. These data indicate that by acting on β1-AR, NA modulates TRPV1 and P/Q-type VGCC and, therefore, motoneuron Ca2+ concentration and the likelihood of ACh synaptic vesicle release (Fig. 2).
(3) Does sympathetic neuron regulation of NMJ transmission decline with aging?
To address this question, we analyzed sciatic-peroneal nerve immunolabeling co-registration and NMJ transmission recordings in a novel mouse ex-vivo preparation. The sympathetic-peroneal nerve/lumbricalis muscle preparation (SPNL) maintains the anatomical complexity of the relationship between the sympathetic and motor nervous systems. This approach unveils the molecular substrate that accounts for the influence of endogenous sympathetic neurons on motoneuron-muscle transmission in young mice and older rodents, providing a unique opportunity to examine whether sympathetic neuron regulation of NMJ transmission declines with age.(Wang et al., 2020a)
Compared to young mice, geriatric mice show no significant change in NMJ-transmission frequency but higher MEPP amplitude and lower EPP amplitude and quantal content.(Wang et al., 2020a) MEPP frequency and amplitude vary according to the muscle examined. In age-matched CBF-1 mice, MEPP frequency decreased in EDL, soleus, and EDC but not gluteus maximus or diaphragm muscles, while MEPP amplitude increased in all but diaphragm.(Banker et al., 1983)
In aging lumbricalis NMJs, sympathetic neuron stimulation does not increase MEPP frequency. Our nerve image co-registration analysis indicates that the strong decline in motoneuron β1-AR expression with aging is the factor limiting its synaptic vesicle release. In contrast to the reported increase in β-AR in the cerebral cortex, hypothalamus,(Meitzen et al., 2013) and hippocampus with aging,(Santulli and Iaccarino, 2013) our data show a significant decline in β1-AR in choline-acetyltransferase and β1- and α2B-AR in tyrosine hydroxylase immunoreactive axons. We proposed that the age-dependent decline in β1-AR expression in sympathetic neurons contributes to diminished NA release.(Wang et al., 2020a)
For functional recordings, we used the SPNL technique to determine whether sympathetic neuron regulation of motoneurons synaptic vesicle release mediated by β1- and α2B-AR is diminished in geriatric mice. However, this approach has some limitations. First, SPNL cannot assess the influence of sympathetic relays from the brain and brainstem nuclei on the intermediolateral column of the spinal cord. Second, it cannot assess the systemic influence of the humoral milieu on the structures involved in neuromuscular transmission. Third, repetitive stimulation might exhaust catecholamine and/or acetylcholine neuron pools. Future experiments in anaesthetized, live mice can overcome these limitations. SNS activation may influence the activity of peripheral targets other than skeletal muscle to modify neuromuscular transmission and muscle innervation. Optogenetic studies targeting specific areas of the SNS should address this question (see below).
Older adults younger than 75 show modest effects of aging on basal SNS activity.(Ng et al., 1994) Above 75, concentrations of basal plasma NA and NA responses to a stressor were higher than did either younger subjects. (Barnes et al., 1982; Esler et al., 1995; Hoeldtke and Cilmi, 1985; Mazzeo et al., 1997; Palmer et al., 1978; Veith et al., 1986) Exaggerated increased basal plasma NA suggests advanced age has specific effects on the sympathoneural and sympathoadrenomedullary components of the SNS.(Pascualya et al., 1999) Both increased release and decreased clearance would account for high NA levels with advanced age.(Pascualya et al., 1999) SNS hyperactivity with aging is associated with ARs desensitization, downregulation, and changes in subtypes expression patterns.(Insel, 1993) Cell-signaling molecules, such as GPRC kinases, are responsible for β-AR phosphorylation, desensitization, and downregulation, (Urasawa et al., 1991) which may contribute to the dysfunction of the target; (Pfleger et al., 2019) for example, the age-dependent impairment of NMJ transmission in response to sympathetic stimulation. β1-AR is among the most studied AR subtypes, probably due to its role in excitation-contraction coupling in normal and diseased hearts. Chronic ischemic heart failure, common in older adults, is associated with decreased β1-AR expression after a period of increased expression that also increases cAMP and protein-kinase A. Phosphorylated GATA4 then mediates the upregulation of miRNA let7, which directly targets ADRB1 mRNA 3’UTR to repress β1-AR. (Du et al., 2017). Whether this mechanism operates in distal axons is unknown. Overall, regulation of β-AR expression in the nervous system and especially the nerve is unclear.
Our data on the age-dependent decline in α2-AR levels are consistent with their diminished responsiveness in prejunctional noradrenergic nerve terminals reported in the cardiovascular system.(Docherty, 2002) However, the mechanism leading to the age-dependent decline in their expression in the spinal nerve remains unknown.
In summary, the age-dependent decline in sympathetic axon β1 and α2B and motoneuron β1-AR accounts for the decline in EPP amplitude and synaptic vesicle quantum content (Fig. 2).
The pharmacological vs the physiological approach to restor SNS influence on NMJ transmission
Maintaining or recovering sympathetic muscle innervation throughout life is undoubtedly preferable to chronic use of AR pharmacological agents. Drug administration cannot mimic the complex, concerted tissue response to endogenous sympathetic neurotransmitters, which depends on the AR subtype’s repertoire and location and the intricate relationship between central and peripheral nervous system relays. Loss of receptor selectivity, tolerance, and tachyphylaxis can also trigger adverse effects. Moreover, pharmaceutical compounds reach stable levels in blood, precluding any adaptation to physiological or pathological challenges. Thus, we cannot prevent or reverse age-related decline in skeletal muscle mass and force until we develop interventions that target the molecular mechanisms governing NMJ instability and muscle denervation.
(4) Does maintenance of sympathetic neurons attenuate aging sarcopenia?
The transcription factor Hand2 points to an intervention that will maintain sympathetic neurons and attenuate sarcopenia with aging
The process by which sympathetic neurons are maintained during development has been amply investigated. (Rohrer, 2011) However, the factors that sustain its integrity into old age are unknown. The differentiation of neural crest cells into NA sympathetic neurons is complex and requires a network of transcription factors. One of them, Hand2, plays a prominent role in adult sympathetic neuron maintenance of NA genes and cell survival as demonstrated by axotomy (Pellegrino et al., 2011) and in conditional Hand2 KO sympathetic neurons. (Stanzel et al., 2016) The Hand2 gene encodes a protein in the basic helix-loop-helix family of transcription factors. Eliminating it in adult sympathetic neurons reduces expression of the genes involved in synapse development and function.(Stanzel et al., 2016) Moreover, in vitro knockdown of Hand2 in differentiated, postmitotic neurons leads to a selective loss of TH and DBH with no effect on generic neuronal genes such as neuron-specific class III beta-tubulin (Tuj1), embryonic lethal, abnormal vision-like RNA-binding protein level 3 (ELAVL3 or HuC) and superior cervical ganglia.(Schmidt et al., 2009) Conditional Hand2 knockout in Hand2-DBHCre mouse embryos caused a selective reduction of TH and a massive reduction of proliferating, DBH-expressing immature neurons.(Schmidt et al., 2009) Despite the detailed examination of Hand2’s function in neurogenesis, and noradrenergic differentiation in embryonic, postnatal, and adult mice,(Rohrer, 2011) analysis of its pivotal role in sympathetic neuron genotype and phenotype in aged mammals is missing and necessary for its potential to attenuate or reverse sarcopenia.
Hand2 expression declines throughout the mouse’s life, but inducing it even at advanced ages preserves the number and size of paravertebral sympathetic ganglia neurons
Hand2 plays a prominent role in adult sympathetic neurons’ adrenergic differentiation and maintenance. (Doxakis et al., 2008; Tsarovina et al., 2010) Figure 3 shows the convergence of a hierarchical network comprised of the bone morphogenetic proteins (BMPs), paired-like homeobox 2b (Phox2b), achaete-scute family BHLH transcription factor 1 (Ascl1), and the insulinoma-associated-1 (Insm1) that regulates its expression. GATA2 and its downstream GATA3 transcription factors are also required for the survival of embryonic and adult sympathetic neurons,(Tsarovina et al., 2010) but their role in old age has not been explored.
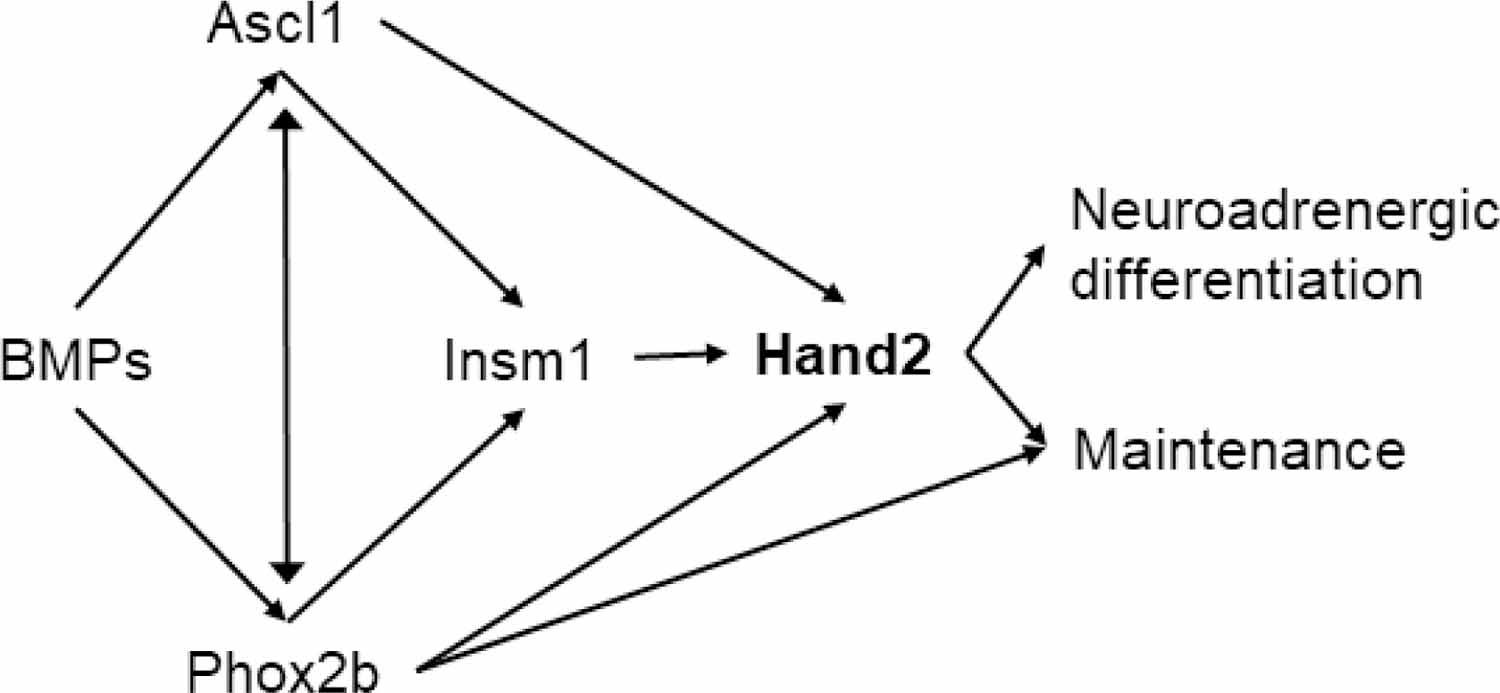
Relationship among the bone morphogenetic protein (BMP)‐ induced transcription factors paired-like homeobox 2b (Phox2b), Achaete-Scute Family BHLH Transcription Factor 1 (Ascl1), Insulinoma-associated 1 (Insm1), and Hand2 (black arrows). Adapted from (Rohrer, 2011). Ascl1: Achaete-scute homolog 1; BMP: bone morphogenetic protein; Hand2: heart and neural crest derivative 2; Insm1: insulinoma associated 1; Phox2b: paired-like homeobox 2b.
Adult nerve injury suppresses Hand2, which directly regulates DBH expression (Rychlik et al., 2005) and, most important, the genes controlling synaptic and neurotransmission functions in adult sympathetic neurons. (Stanzel et al., 2016) Based on the reported role of the transcription factors supporting developing and adult autonomic neurons,(Stanzel et al., 2016) we examined whether inducing HAND2 expression exclusively in sympathetic neurons would attenuate (a) motor denervation, (b) impaired NMJ transmission, and (c) loss of muscle mass and function in old mice. We delivered a viral vector carrying Hand2 expression or an empty vector (EV) exclusively to sympathetic neurons. Since Hand2 expression is restricted to the peripheral SNS, (Hendershot et al., 2008) we ruled out any influence of central sympathetic nuclei on our measures.
Neurons from 22-month-old EV-treated mice were not immunoreactive to Hand2 antibody probably because they had less than 20 percent of the transcript recorded at postnatal day-5.(Rodrigues et al., 2020) Sympathetic neuron hypermethylation may account, at least partially, for this age-dependent decrease in Hand2 gene transcription, since CpG islands, but not C combined with any other nucleotide (CH or CHH) exhibit hypermethylation in paravertebral sympathetic ganglia neuron with aging. (Rodrigues et al., 2020) Epigenetic silencing of Hand2 has been reported in other territories in older adults, for instance in the development of endometrial cancer, which occurs in older women. (Jones et al., 2013) Hand2 undergoes age-associated DNA hypermethylation in epithelial tissues.(Maegawa et al., 2010) The mouse intestine, a tissue densely innervated by the SNS, shows a high rate of Hand2 hypermethylation as a function of age, suggesting that Hand2 can be hypermethylated in other cells as well. (Maegawa et al., 2010)
Sustained Hand2 expression in sympathetic neurons in old mice preserves ganglia sympathetic neurons size to a significant extent, which indicates that Hand2 ameliorates the loss of ganglia neurons with aging. (Stanzel et al., 2016) Conditional deletion of Hand2 reveals its critical functions in neurogenesis during development (Hendershot et al., 2008) and adulthood. (Stanzel et al., 2016) Preserving the number and size of sympathetic neurons in Hand2-treated mice extends and enriches the complexity of sympathetic muscle innervation with aging.
The increase in phosphorylated PKA RI and mechanistic target of rapamycin complex 1 (mTORC1) with Hand2 increases phosphorylation of the cAMP-response element binding protein CREB modulator- CREM, which translocates to the nucleus. Both CREB and CREM, bind a specific cis element - cAMP-response element (CRE) - in the promoter region of skeletal muscle target genes (Zheng et al., 2002). In the Hand2-treated mice, upregulation of Crtc3, a member of the CREB regulated transcription co-activator gene family, is consistent with activation of cAMP-responsive genes. CREM, a top upstream regulator of genes controlled by sympathetic neurons expressing Hand2 in old age. CREM targets include the TH and dHand, two genes essential for SN function and maintenance (Rauen et al., 2013). In summary, low levels of PKA RI phosphorylation (Xu et al., 2017), together with genomic hypermethylation may account for the age-dependent decrease in Hand2 gene transcription.
Hand2 increases skeletal muscle mass and force and whole-body strength but not spontaneous mobility or endurance
The increase in muscle mass in Hand2- compared to EV-treated mice was associated with enhanced muscle strength, which we attribute to the increase in cross-sectional area (CSA) of type-II fibers in extensor digitorum longus (EDL) muscle, and type-I and type-II fibers in soleus muscle.(Rodrigues et al., 2020)
In the EV- compared to the Hand2-treated mice, electrical stimulation of distal peroneal nerves resulted in unsustained and weaker lumbricalis muscle peak force. However, this effect was more pronounced in response to muscle activation through the nerve. These data indicate that in addition to muscle sympathetic innervation, aging impairs motor innervation and NMJ transmission, and Hand2 expression in sympathetic neurons rescues them significantly. (Rodrigues et al., 2020)
Sympathetic denervation of various target organs
In old mice, we found that blood vessels were still surrounded by sympathetic axons, but their projections toward myofibers and NMJs were fewer, depriving the muscle of the dense sympathetic network observed in young mammals.(Rodrigues et al., 2018; Rodrigues et al., 2020; Straka et al., 2018) The skeletal muscle does not seem to be the only organ progressively losing sympathetic innervation density and complexity, since blood vessels and the heart have been reported to exhibit marked deprivation of sympathetic axons with aging. (Brown et al., 2019; Li et al., 2003; Rengo et al., 2016) The pulmonary nervous system consists of afferent and efferent nerve fibers that help to maintain organ homeostasis.(Brandenberger and Mühlfeld, 2017) In the lower airways, aging is associated with a reduction in noradrenergic innervation of the bronchial tree (Ricci et al., 1997) and a decrease in vasoactive intestinal peptide concentration and nerve fiber density.(Geppetti et al., 1988) Evidence for a decrease in sympathetic control of intestinal function in aged rodents has been reported,(Baker et al., 1991) and the timing aligns with the onset and rate of myenteric cell loss.(Cowen et al., 2000; El-Salhy et al., 1999; Phillips and Powley, 2001; Phillips and Powley, 2007) Noradrenergic networks are abundant in young adult rats, but in aged rats, noradrenergic innervation is less dense, and splenic NA levels are significantly lower. A relationship between diminished noradrenergic innervation and reduced immune responsiveness in aging mammals has been proposed.(Felten et al., 1987)
The mechanism by which organs lose sympathetic axon terminals is incompletely understood. During development, target tissues secrete trophic factors to regulate axon branching at sympathetic nerve terminals, an essential step in forming functional connections. Compartmentalized neuronal cultures revealed that the autocrine Wnt5a-(retinoic acid-related orphan receptor [ROR]) signaling loop directs sympathetic axon branching during target innervation. (Ryu et al., 2013) Although Wnt5a induced β-catenin nuclear translocation in vascular smooth muscle cells (VSMCs) from young and old mice, CREB phosphorylation and Wnt1-inducible signaling pathway protein-1 (WISP-1) upregulation did not occur in old VSMCs. Wnt5a-mediated survival was lost with aging due to impaired CREB activation and WISP-1 regulation. Better understanding of the effect of age on Wnt signaling may identify targets to promote VSMC survival in elderly patients.(Brown et al., 2019) Due to the relevance of skeletal muscle sympathetic innervation, we tested whether maintaining postganglionic sympathetic neurons by Hand2 expression prevents or attenuates NMJ transmission and muscle innervation.
Hand2 expression enhances NMJ transmission and muscle innervation
Sympathetic neuron Hand2 enhances spontaneous and evoked NMJ transmission and prevents myofiber motor and sympathetic denervation. It also preserves the complexity of muscle sympathetic innervation and its proximity to the NMJ. Fragmentation and simplification of the postterminals are hallmarks of age-dependent deterioration of skeletal muscle innervation, (Li and Thompson, 2011) while sustained Hand2 expression by sympathetic neurons preserves their molecular integrity and morphology. Hand2-treated mice had fewer small postterminal fragments than the EV group and showed an approximately five-fold decrease when the number of fragments was normalized to the muscle area. The wider range of postterminal fragment sizes in the Hand2 group indicates that, to a significant degree, muscle sympathetic innervation ameliorates fragmentation.
Hand2 significantly preserves skeletal muscle sympathetic innervation, as shown by (a) higher TH levels in nerve and muscle, detected by immunoblot; (b) longer muscle sympathetic axon path; and the shorter distance between sympathetic neurons and NMJs, confirmed by immunohistochemistry. NMJ pre- and postterminal co-localization and quantification of neurofilament (NF) path length per muscle area shows that the preservation of sympathetic innervation enhances myofiber motor innervation. The mechanism underlying preservation of muscle sympathetic and motor innervation is uncertain; however, low protein phosphatase-2 (PP2A), and protein phosphatase-1 (PP1) levels and more NF-heavy chain (NFH-), NF-medium chain (NFM-), and NF-light chain (NFL) subunit phosphorylation indicate that NF phosphorylation plays a role in stabilizing muscle motor innervation in Hand2-treated mice. This conclusion is further supported by confocal and electron microscopy studies on muscle sympathetic ablation, which show compromised NF phosphorylation and disorganized axon NF and microtubules.(Rodrigues et al., 2020; Rodrigues et al., 2019)
Additionally, Hand2 upregulates the genes encoding critical components of NMJ and myofiber innervation, including Nrcam, a neural cell-adhesion molecule, its product, NCAM, which is a marker of muscle denervation/reinnervation, (Messi et al., 2016) and Nrxn1, a presynaptic membrane cell-adhesion molecule that primarily binds to neuroligins, forming a complex required for efficient neurotransmission(Hadley et al., 2006). Hand2 also upregulates the microtubule-associated protein-2 (MAP2) gene, which may stabilize the microtubules against depolimerization, synapse differentiation inducing 1 like (Sindig1l), which plays a role in the shaping of synaptic specificity,(Cadwell et al., 2016) and the GDNF receptor Gfra1, expressed at myelinated nerves and NMJ. Both GDNF and Gfra1 play a crucial role in muscle innervation and GDNF signaling. (Hase et al., 1999; Henderson et al., 1994) (Rodrigues et al., 2020) These results indicate that Hand2 expression enhances NMJ transmission and muscle innervation in the old.
G protein-coupled receptor (GPCR) signaling plays a pivotal role in sympathetic neuron-mediated myofiber maintenance
The GPCR constitute the largest family of membrane receptors. It is responsible for cell signaling upon binding to hormones, neurotransmitters, or ions. Notably, many pharmacological agents target these receptors, stressing their involvement in pathophysiological conditions and therapeutics.(Rosenbaum et al., 2009) In contrast to stimulating Gs, the inhibitory subunit Gαi2 isoform blocks adenylate cyclase activation and cAMP production. Physiological or pathological circumstances that decrease levels of sympathetic neurotransmitter or Gαi2 expression prevents the myogenin- and MuRF1-mediated pro-cachectic action of tumor necrosis factor alpha (TNF-α) and myofiber atrophy. (Minetti et al., 2014) Minetti and coworkers showed that Gαi2 induces muscle hypertrophy by promoting mTOR and protein synthesis in an Akt-independent mechanism, while inhibiting atrophy in response to TNF-α. Ablation of muscle sympathetic innervation decreases muscle NA,(Silveira et al., 2014) and Gαi2 protein levels leading to muscle atrophy. (Rodrigues et al., 2018) The model of muscle sympathetic ablation shows downregulation of Gαi2, which activates the Hdac4-myogenin-MyoD cascade to raise MuRF1 levels and increase AChR destabilization.(Rodrigues et al., 2019) Note that both experimental sympathetic ablation and aging can cause vasculature denervation, which might influence the deterioration of muscle structure and function. However, muscle flow recordings have shown that the effect of epinephrine on neuromuscular transmission is independent of concomitant vascular changes produced by the catecholamine. (Bowman and Raper, 1966) These data indicate that catecholamines trigger intramyocellular signaling independently of their vasoactive function.
Sympathetic neuron Hand2 prevents Gαi2 downregulation with aging
We found that Hand2 expression in sympathetic neurons prevents the decrease in Gαi2 levels with aging and activation of the signaling cascade that has been associated with muscle motor denervation.(Minetti et al., 2014) It also lowers Hdac4 concentration to improve motor-axon function. (Cohen et al., 2007; Moresi et al., 2010) Hdac4 mediates skeletal muscle response to denervation, suggesting its inhibition as a target for treating neurogenic muscle atrophy. However, studies in mutant mice where HDAC4 is specifically deleted in the skeletal muscle,(Pigna et al., 2018) show that it preserves skeletal muscle structure following long-term denervation, suggestging that its role in muscle denervation is more complex than previously thought. It might involve oxidative stress, the ubiquitin-proteasome system, and autophagy.(Pigna et al., 2018)
Hand2 also downregulates myogenin and myoD, both associated with denervation in gastrocnemius and tibialis anterior muscles. Myogenin and myoD genes are regulated by electrical activity,(Eftimie et al., 1991; Tang and Goldman, 2006). Downregulating these genes reduces MuRF1 and atrogin-1. MuRF1 is expressed in both type‐ I and type‐ II fibers, but predominantly the latter; it is highly enriched in the postterminal; interacts with AChR in endocytic structures; (Rudolf et al., 2013) and participates in muscle trophism and maintenance. (Moriscot et al., 2010) Consistently, Hand2-induced preservation of muscle sympathetic innervation downregulates both MuRF1 and atrogin and prevents AChR endocytosis.
In old mice, the PKA RIα subunit is largely removed from the NMJ, while PKA RIIα and RIIβ are enriched there (Xu et al., 2017). Induced sustained levels of Hand2 in sympathetic neurons, like other interventions to counter muscle denervation (Xu et al., 2017), upregulates PKA RI in the TA muscle, and downregulates PKA RII and the PKA RII/PKA RI ratio in both GA and TA muscles. These results support the hypothesis that muscle sympathetic innervation prevents activation of the signaling cascade reported in skeletal muscle denervation,(Moresi et al., 2010) by maintaining Gαi2 expression.
Mechanisms by which Hand2 prevents age-related decline in muscle mass and force. Role of Akt, atrogins, inflammation, mTORC1, and autophagy (Figure 4)
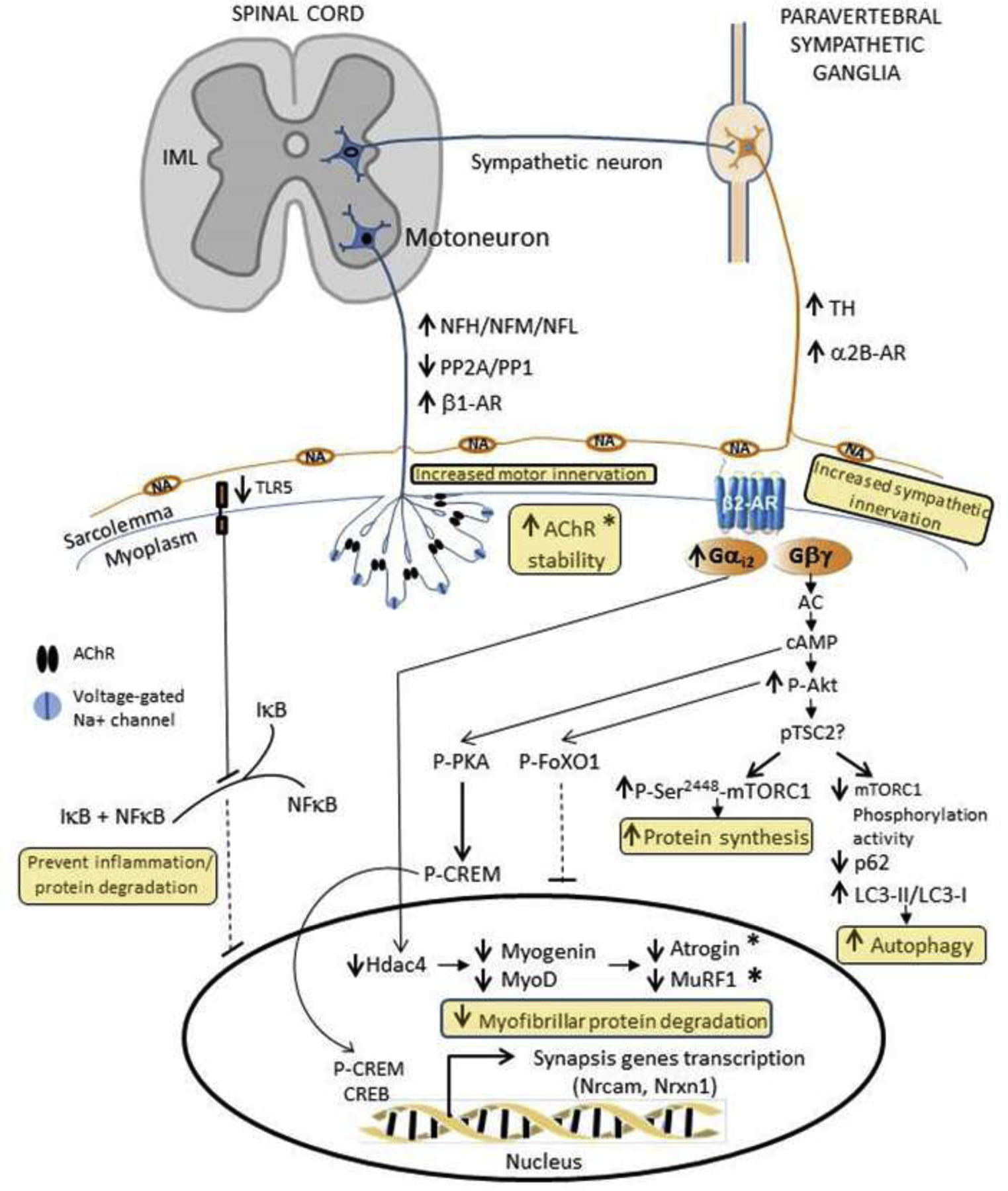
Selective expression of Hand2 in the mouse peripheral sympathetic system from middle- through old-age increases muscle mass and force by (a) regulating skeletal muscle sympathetic and motor innervation; (b) improving AChR stability and NMJ transmission; (c) preventing inflammation and myofibrillar protein degradation; (d) increasing autophagy; and (e) probably enhancing protein synthesis. (Rodrigues et al., 2020). AChR: acetylcholine receptor; CREB: cAMP response element-binding protein; CREM: cAMP Responsive Element Modulator; FoXO1: Forkhead box protein O1; IκB: inhibitor of nuclear factor kappa B; IML: intermediolateral column; LC-I/LC-II: microtubule-associated protein light chain; mTOR: the mechanistic target of rapamycin; NA: noradrenaline; NFκB: nuclear factor kappa-light-chain-enhancer of activated B cells; NFH: neurofilament heavy chain; NFL: neurofilament light polypeptide; NFM: neurofilament medium chain; Nrcam: Neuronal cell adhesion molecule; Nrxn1: neurexin-1-alpha; p62: 62kDa protein; PKA: protein kinase A; TH: tyrosine hydroxylase; TSC2: tuberous sclerosis complex 2; TLR5: Toll-like receptor 5.
The protein kinase B (a.k.a. Akt) promotes myotube hypertrophy by stimulating protein synthesis through mTOR activity while inhibiting the forkhead box FoxO to reduce MuRF1 abundance, preventing myofibrillar protein turnover.(Rommel et al., 2001) Hand2 treatment increases muscle Akt phosphorylation, a critical step in the activation of the noncanonical β-AR pathway, which leads to FoXO1 phosphorylation and exclusion from the nucleus and downregulation of MuRF1 and atrogin/MAFbx. (Rodrigues et al., 2020) This mechanism, together with downregulation of the Hdac4-myogenin-MyoD-atrogin/MuRF1 signaling cascade (see above), reduces atrogin expression to prevent muscle atrophy.(Ryall et al., 2008)
Activation of the transcription factor nuclear factor kappa B (NF-κB) is a fulcrum in the processes that mediate muscle atrophy. The significance of NF-κB as a key regulator of muscle atrophy has been emphasized by several in vivo studies, which have demonstrated that NF-κB- targeted therapies can abrogate muscle atrophy.(Thoma and Lightfoot, 2018) NFκB signaling plays a role in age-related skeletal muscle atrophy probably by activating the senescence-associated secretory phenotype.(Salminen et al., 2009) Moreover, Hand2 decreased the inhibitor IκB and NFκB phosphorylation. (Rodrigues et al., 2020) These results support our RNA-sequencing analysis showing that Hand2-induced toll-like receptor 5 (Tlr5) downregulation decreases IκB activation, leading to nuclear exclusion of NFκB and preventing activation of the canonical pro-inflammatory pathway. Consistently, downregulation of TNF-related-weak-inducer-of-apoptosis (TWEAK) and Toll-like receptor-1 (TLR-1) and interleukin (IL) 16, which play roles in the innate immune system and the cell cycle, respectively, contribute to SNS regulation of inflammation and immune-system homeostasis.(Révész et al., 2014) Cytokines downregulate Hand2 in cultured sympathetic neurons, while Hand2 overexpression rescues the noradrenergic phenotype. These observations support a third mechanism for decreased Hand2 transcription with aging, in addition to reduced levels of PKA RI (Xu et al., 2017) and Hand2 genomic DNA hypermethylation.
Rapamycin-induced mammalian target of rapamycin complex 1 (mTORC1) inhibition prevents age-related muscle loss, while its chronic activation advances muscle damage and loss,(Tang et al., 2019) inhibiting constitutive and starvation-induced autophagy in skeletal muscle. (Castets et al., 2013) We found in both gastrocnemius and tibialis anterior muscles, that Hand2 treatment increases mTORC1 phosphorylation at serine 2448, fine-tuning its activity at the “repressor domain.(Chiang and Abraham, 2005; Figueiredo et al., 2017) Deleting this site strongly activates mTORC1.(Sekulic et al., 2000) Increased phosphorylation at the repressor domain prevents Unc-51-like kinase (ULK) and autophagy-related-13 (ATG13) phosphorylation, activating autophagy (Laplante and Sabatini, 2012).
Does increased macroautophagy improve skeletal muscle mass and/or function in old mice? One common feature of all age-related changes at the tissue level is the accumulation of damage and harmful modifications in DNA, proteins, lipids, and cellular organelles; autophagic efficiency declines over time, and intracellular waste products amass.(Salminen et al., 2009) Robust genetic evidence across species supports the inhibition of autophagy in aging. (Carnio et al.; Rubinsztein et al., 2011) Analysis of muscle autophagy showed lower p62 and higher ATG-7 levels and LC3-I lipidation in the Hand2 group than the EV group. Atg7 ablation induces NMJ degeneration and precocious aging (Carnio et al., 2014), which suggests that high ATG-7 levels are required to improve muscle autophagy. Autophagy selectively degrades the LC3-binding adaptor p62 protein (sequestosome-1 [SQSTM1]),(Ichimura et al., 2008) and since a drop in p62 is associated with activation of the autophagic process, it serves as a readout of autophagic flux, together with LC3-I conversion to LC3-II.(Bjorkoy et al., 2005; Seibenhener et al., 2004) Non-significant changes in lysosome-associated membrane protein 2 (Lamp2) and heat-shock cognate protein of 70kDa (HSC70) levels indicate that macroautophagy, but not chaperone-mediated autophagy, is activated by sustained Hand2 expression in sympathetic neurons with aging. Note that direct stimulation of sympathetic neurons activates muscle postsynaptic β2-AR, cAMP production, and import of the transcriptional co-activator peroxisome proliferator-activated receptor γ-coactivator 1α (PPARGC1A) into the myonucleus,(Khan et al., 2016) which increases autophagy.(Vainshtein et al., 2015)
In summary, preventing decline in peripheral sympathetic neuron Hand2 expression with aging attenuates sympathetic and motor muscle denervation and aging sarcopenia. (Rodrigues et al., 2020)
(5) Do central sympathetic neuron relays influence sympathetic and motor muscle innervation?
The studies reviewed above indicate that activation of peripheral sympathetic neurons increase NMJ transmission. However, we must still define the specific neuronal groups in the peripheral and central nervous system that account for SNS influence on NMJ transmission; for example, whether or how postganglionic sympathetic neurons regulate synaptic vesicle release in vivo or central autonomic relays regulate NMJ transmission. Previous studies have associated the pontine locus coeruleus (LC) (a.k.a. A6 nucleus) with human mobility, motility, and skeletal muscle physiology.(Buchman et al., 2012; Del Tredici and Braak, 2013; Dobbins and Feldman, 1994; Jacobs et al., 1991; Robinson, 1978; Xiang et al., 2014) A series of experiments in our laboratory focused on lumbar postganglionic sympathetic neurons, and the LC, a subdivision of which projects to preganglionic neurons in the spinal cord intermediolateral (IML) column to address this issue. Combining optogenetics (a technique that involves the use of light to control neuron activity that have been genetically modified to express light-sensitive ion channels) with NMJ transmission recordings provided a unique opportunity to determine the precise role of postganglionic sympathetic neurons and LC neurons in NMJ transmission in adult living mice. (Rodrigues et al., 2020)
We crossed Ai32(RCL-ChR2(H134R/EYFP))(Madisen et al., 2012) and TH-Cre (Savitt et al., 2005) to create a mouse model that would express channelrodhopsin-2 (ChR2) in the central and peripheral noradrenergic neurons. We concluded that in vitro and in living mice sympathetic axon optostimulation, mediated by β1-ARs, enhances NMJ transmission and LC does not, despite its projections to the IML, (Rodrigues et al., 2020) perhaps because they do not establish en passant synapse with preganglionic sympathetic neurons. Confocal z-stack projections of sections through the spinal cord showed that the injected AAV2/rh8-PRSx8-EGFP-W viral vector appears at both the dorsal horn and the IML. (Bruinstroop et al., 2012) Also, we stimulated LC at frequencies below and above 5Hz with no obvious response, which indicates that by innervating the muscle spindle, (Radovanovic et al., 2015) LC modulates posture, (Carter et al., 2010) but not NMJ transmission.
Detailed analysis of central neuroanatomical circuitries show that several brainstem noradrenergic regions project to the IML, including the noradrenergic bulbospinal A1 and A2 areas (Rodovalho et al., 2020) and pontine A5, A6, and A7. (Bruinstroop et al., 2012) The functional relevance of these CNS projections for NMJ transmission and muscle motor innervation remains unknown. Elucidating the functional role of brainstem sympathetic neuron projections to preganglionic noradrenergic neurons will help to define the coordinated response of the visceral and neuromuscular system to physiological and pathological challenges.
Conclusions and future directions
The SNS regulates several primary synaptic and non-synaptic events. Inducing Hand2 expression in sympathetic neurons from middle-aged mice improves their NMJ transmission, myofiber innervation, muscle mass, and function maintenance as they age. Despite recent progress in our understanding of the process by which the SNS regulates skeletal muscle structure and function with aging, several questions remain. Hand2 is critical for sympathetic neurons maintenance in adult mice, but its expression is regulated by transcription factors, such as BMPs, ASCL1, Phox2b, and Insm1,(Rohrer, 2011) and their expression levels and function have not been examined in aging organisms. Also, despite the beneficial impact of Hand2 induced expression on muscle innervation, we do not know whether Hand2 expression induced for a longer time, and up to very old age (geriatric) will still ameliorate sarcopenia. Treated middle-aged mice should be examined not only 6-months, but 10–12 months after Hand2- or EV-treatment.
Whether mechanisms other than hypermethylation influence Hand2 expression in aging sympathetic neurons remains unknown. Although some of these mechanisms are discussed above, the potential role of the recently identified enhancer RNAs (eRNAs) should be investigated. eRNAs are a novel class of short, noncoding RNAs transcribed from active enhancer regions in the genome. They regulate downstream genes promoting DNA looping/interactions, regulating chromatin accessibility, and interfering in transcription, which is clinically relevant.(Li et al., 2014; Li et al., 2016; Mora et al., 2016)
We do not know whether the age-dependent decline in central autonomic nucleus composition and/or function accounts for skeletal motor denervation and sarcopenia. A decrease in LC neuron density is associated with impaired mobility in older adults, which indicates that central sympathetic relays may play a critical role in physical activity.(Buchman et al., 2012) Our research shows that postganglionic, but not LC neurons regulate NMJ transmission. Whether other brainstem noradrenergic neuron groups more densely projected to the IML influence s mammalians’ motility is an open question for future research on the mechanisms of aging sarcopenia.
Recent findings can be translated to human sarcopenia. Non-pathogenic viral vectors with long-lasting expression, recently tested in rodents, could be used for safe, efficient gene delivery in humans in the near future.(Rodrigues et al., 2020) Nanomaterials or lipid micelles are alternative approaches to deliver Hand2 to ganglionic sympathetic neurons.
Acknowledgments
The National Institutes of Health grants R01AG057013 and R01AG057013–02S1, and the Wake Forest Claude D. Pepper Older Americans Independence Center (P30-AG21332) supported this work and the original research published by the Delbono laboratory.
Footnotes
Publisher's Disclaimer: This is a PDF file of an unedited manuscript that has been accepted for publication. As a service to our customers we are providing this early version of the manuscript. The manuscript will undergo copyediting, typesetting, and review of the resulting proof before it is published in its final form. Please note that during the production process errors may be discovered which could affect the content, and all legal disclaimers that apply to the journal pertain.
Financial interest: The author declares that he has no conflicts of interest.
Conflicts of interest
The authors declare that they have no conflicts of interest.
References
- Andersson AM, Olsen M, Zhernosekov D, Gaardsvoll H, Krog L, Linnemann D, Bock E, 1993. Age-related changes in expression of the neural cell adhesion molecule in skeletal muscle: a comparative study of newborn, adult and aged rats. Biochem J 290 (Pt 3), 641–648. [PMC free article] [PubMed] [Google Scholar]
- Askmark H, Eeg-Olofsson K, Johansson A, Nilsson P, Olsson Y, Aquilonius S, 2001. Parkinsonism and neck extensor myopathy: A new syndrome or coincidental findings? Archives of Neurology 58, 232–237. [PubMed] [Google Scholar]
- Baker DM, Watson SP, Santer RM, 1991. Evidence for a decrease in sympathetic control of intestinal function in the aged rat. Neurobiology of Aging 12, 363–365. [PubMed] [Google Scholar]
- Balice-Gordon RJ, 1997. Age-related changes in neuromuscular innervation. Muscle & Nerve S5, S83–S87. [PubMed] [Google Scholar]
- Banker BQ, Kelly SS, Robbins N, 1983. Neuromuscular transmission and correlative morphology in young and old mice. J Physiol 339, 355–377. [PMC free article] [PubMed] [Google Scholar]
- Barker D, Saito M, 1981. Autonomic innervation of receptors and muscle fibres in cat skeletal muscle. Proc R Soc Lond B Biol Sci 212, 317–332. [PubMed] [Google Scholar]
- Barnes RF, Raskind M, Gumbretch G, Halter JB, 1982. The effects of age on the plasma catecholamine response to mental stress in man. The Journal of Clinical Endocrinology & Metabolism 54, 64–69. [PubMed] [Google Scholar]
- Beitzel F, Sillence MN, Lynch GS, 2007. beta-Adrenoceptor signaling in regenerating skeletal muscle after beta-agonist administration. Am J Physiol Endocrinol Metab 293, E932–940. [PubMed] [Google Scholar]
- Bergmeister KD, Gröger M, Aman M, Willensdorfer A, Manzano-Szalai K, Salminger S, Aszmann OC, 2016. Automated muscle fiber type population analysis with ImageJ of whole rat muscles using rapid myosin heavy chain immunohistochemistry. Muscle & Nerve 54, 292–299. [PubMed] [Google Scholar]
- Bezakova G, Lomo T, 2001. Muscle activity and muscle agrin regulate the organization of cytoskeletal proteins and attached acetylcholine receptor (AchR) aggregates in skeletal muscle fibers. J Cell Biol 153, 1453–1463. [PMC free article] [PubMed] [Google Scholar]
- Biral D, Kern H, Adami N, Boncompagni S, Protasi F, Carraro U, 2008. Atrophy-resistant fibers in permanent peripheral denervation of human skeletal muscle. Neurological Research 30, 137–144. [PubMed] [Google Scholar]
- Bjorkoy G, Lamark T, Brech A, Outzen H, Perander M, Overvatn A, Stenmark H, Johansen T, 2005. p62/SQSTM1 forms protein aggregates degraded by autophagy and has a protective effect on huntingtin-induced cell death. J Cell Biol 171, 603–614. [PMC free article] [PubMed] [Google Scholar]
- Blasco A, Gras S, Mòdol-Caballero G, Tarabal O, Casanovas A, Piedrafita L, Barranco A, Das T, Pereira SL, Navarro X, Rueda R, Esquerda JE, Calderó J, 2020. Motoneuron deafferentation and gliosis occur in association with neuromuscular regressive changes during ageing in mice. J Cachexia Sarcopenia Muscle 6, 1628–1660. [PMC free article] [PubMed] [Google Scholar]
- Bloemberg D, Quadrilatero J, 2012. Rapid determination of myosin heavy chain expression in rat, mouse, and human skeletal muscle using multicolor immunofluorescence analysis. PLoS One 7, e35273. [PMC free article] [PubMed] [Google Scholar]
- Bodine SC, Latres E, Baumhueter S, Lai VK, Nunez L, Clarke BA, Poueymirou WT, Panaro FJ, Na E, Dharmarajan K, Pan ZQ, Valenzuela DM, DeChiara TM, Stitt TN, Yancopoulos GD, Glass DJ, 2001. Identification of ubiquitin ligases required for skeletal muscle atrophy. Science 294, 1704–1708. [PubMed] [Google Scholar]
- Boeke J, 1909. Ueber eine aus marklosen Fasern hervorgehende zweite Art von hypolemmalen Nervenendplatten bei den quergestreiften Muskelfasern der Vertebraten. Anat. Anz 35, 481–484. [Google Scholar]
- Bonaldo P, Sandri M, 2013. Cellular and molecular mechanisms of muscle atrophy. Disease Models & Mechanisms 6, 25–39. [PMC free article] [PubMed] [Google Scholar]
- Bonilla HJ, Messi ML, Sadieva KA, Hamilton CA, Buchman AS, Delbono O, 2020. Semiautomatic morphometric analysis of skeletal muscle obtained by needle biopsy in older adults. GeroScience 6, 1431–1443. [PMC free article] [PubMed] [Google Scholar]
- Bowman WC, Raper C, 1966. Effects of Sympathomimetic Amines on Neuromuscular Transmission. British Journal of Pharmacology and Chemotherapy 27, 313–331. [PMC free article] [PubMed] [Google Scholar]
- Brandenberger C, Mühlfeld C, 2017. Mechanisms of lung aging. Cell and Tissue Research 367, 469–480. [PubMed] [Google Scholar]
- Briguet A, Courdier-Fruh I, Foster M, Meier T, Magyar JP, 2004. Histological parameters for the quantitative assessment of muscular dystrophy in the mdx-mouse. Neuromuscul Disord 14, 675–682. [PubMed] [Google Scholar]
- Brooks SV, Faulkner JA, 1994. Skeletal muscle weakness in old age: underlying mechanisms. Medicine & Science in Sports & Exercise 26, 432–439. [PubMed] [Google Scholar]
- Brown BA, Connolly GM, Mill CEJ, Williams H, Angelini GD, Johnson JL, George SJ, 2019. Aging differentially modulates the Wnt pro-survival signalling pathways in vascular smooth muscle cells. Aging Cell 18, e12844. [PMC free article] [PubMed] [Google Scholar]
- Bruinstroop E, Cano G, Vanderhorst VGJM, Cavalcante JC, Wirth J, Sena-Esteves M, Saper CB, 2012. Spinal projections of the A5, A6 (locus coeruleus), and A7 noradrenergic cell groups in rats. The Journal of Comparative Neurology 520, 1985–2001. [PMC free article] [PubMed] [Google Scholar]
- Buchman AS, Nag S, Shulman JM, Lim AS, VanderHorst VG, Leurgans SE, Schneider JA, Bennett DA, 2012. Locus coeruleus neuron density and parkinsonism in older adults without Parkinson’s disease. Mov Disord 27, 1625–1631. [PMC free article] [PubMed] [Google Scholar]
- Buller AJ, Eccles JC, Eccles RM, 1960a. Differentiation of fast and slow muscles in the cat hind limb. Journal of Physiology 150, 399–416. [PMC free article] [PubMed] [Google Scholar]
- Buller AJ, Eccles JC, Eccles RM, 1960b. Interactions between motoneurones and muscles in respect of the characteristic speeds of their responses. Journal of Physiology 150, 417–439. [PMC free article] [PubMed] [Google Scholar]
- Cadwell CR, Palasantza A, Jiang X, Berens P, Deng Q, Yilmaz M, Reimer J, Shen S, Bethge M, Tolias KF, Sandberg R, Tolias AS, 2016. Electrophysiological, transcriptomic and morphologic profiling of single neurons using Patch-seq. Nat Biotechnol 34, 199–203. [PMC free article] [PubMed] [Google Scholar]
- Cano-Jaimez M, Pérez-Sánchez F, Milán M, Buendía P, Ambrosio S, Fariñas I, 2010. Vulnerability of peripheral catecholaminergic neurons to MPTP is not regulated by α- synuclein. Neurobiology of Disease 38, 92–103. [PubMed] [Google Scholar]
- Card JP, Enquist LW, 2012. Use and Visualization of Neuroanatomical Viral Transneuronal Tracers, in: Badoer E (Ed.), Visualization Techniques: From Immunohistochemistry to Magnetic Resonance Imaging Humana Press, Totowa, NJ, pp. 225–268. [Google Scholar]
- Carlson BM, Borisov AB, Dedkov EI, Khalyfa A, Kostrominova TY, Macpherson PC, Wang E, Faulkner JA, 2002. Effects of long-term denervation on skeletal muscle in old rats. J Gerontol A Biol Sci Med Sci 57, B366–374. [PubMed] [Google Scholar]
- Carnio S, LoVerso F, Baraibar Martin A., Longa E, Khan Muzamil M., Maffei M, Reischl M, Canepari M, Loefler S, Kern H, Blaauw B, Friguet B, Bottinelli R, Rudolf R, Sandri M, 2014. Autophagy Impairment in Muscle Induces Neuromuscular Junction Degeneration and Precocious Aging. Cell Reports 8(5), 1509–21. [PMC free article] [PubMed] [Google Scholar]
- Carter ME, Yizhar O, Chikahisa S, Nguyen H, Adamantidis A, Nishino S, Deisseroth K, de Lecea L, 2010. Tuning arousal with optogenetic modulation of locus coeruleus neurons. Nat Neurosci 13, 1526–1533. [PMC free article] [PubMed] [Google Scholar]
- Carter WJ, Dang AQ, Faas FH, Lynch ME, 1991. Effects of clenbuterol on skeletal muscle mass, body composition, and recovery from surgical stress in senescent rats. Metabolism 40, 855–860. [PubMed] [Google Scholar]
- Castets P, Lin S, Rion N, Fulvio S, Romanino K, Guridi M, 2013. Sustained activation of mTORC1 in skeletal muscle inhibits constitutive and starvation-induced autophagy and causes a severe, late-onset myopathy. Cell Metab 17, 731–744. [PubMed] [Google Scholar]
- Chiang GG, Abraham RT, 2005. Phosphorylation of mammalian target of rapamycin (mTOR) at Ser-2448 is mediated by p70S6 kinase. J Biol Chem 280, 25485–25490. [PubMed] [Google Scholar]
- Chin SO, Rhee SY, Chon S, Hwang Y-C, Jeong I-K, Oh S, Ahn KJ, Chung HY, Woo J. t., Kim S-W, Kim J-W, Kim YS, Ahn H-Y, 2013. Sarcopenia Is Independently Associated with Cardiovascular Disease in Older Korean Adults: The Korea National Health and Nutrition Examination Survey (KNHANES) from 2009. PLOS ONE 8, e60119. [PMC free article] [PubMed] [Google Scholar]
- Choi SJ, Shively CA, Register TC, Stehle J, High K, Ip E, Feng X, Kritchevsky SB, Nicklas BJ, Delbono O, 2012. Force Generation Capacity of Single Muscle Fibers of Vastus Lateralis Declines with Age and Correlates with Physical Function in African Green Vervet Monkeys. Journal of Gerontology. Biological Sciences 68(3), 258–67. [PMC free article] [PubMed] [Google Scholar]
- Cohen TJ, Waddell DS, Barrientos T, Lu Z, Feng G, Cox GA, Bodine SC, Yao TP, 2007. The histone deacetylase HDAC4 connects neural activity to muscle transcriptional reprogramming. J Biol Chem 282, 33752–33759. [PubMed] [Google Scholar]
- Cowen T, Johnson R, Soubeyre V, Santer RM, 2000. Restricted diet rescues rat enteric motor neurones from age related cell death. Gut 47, 653–660. [PMC free article] [PubMed] [Google Scholar]
- Cuervo AM, Palmer A, Rivett AJ, Knecht E, 1995. Degradation of proteasomes by lysosomes in rat liver. Eur J Biochem 227, 792–800. [PubMed] [Google Scholar]
- de Waegh SM, Lee VM, Brady ST, 1992. Local modulation of neurofilament phosphorylation, axonal caliber, and slow axonal transport by myelinating Schwann cells. Cell 68, 451–463. [PubMed] [Google Scholar]
- DeAndrade J, Pedersen M, Garcia L, Nau P, 2018. Sarcopenia is a risk factor for complications and an independent predictor of hospital length of stay in trauma patients. Journal of Surgical Research 221, 161–166. [PubMed] [Google Scholar]
- Del Tredici K, Braak H, 2013. Dysfunction of the locus coeruleus-norepinephrine system and related circuitry in Parkinson’s disease-related dementia. J Neurol Neurosurg Psychiatry 84, 774–783. [PubMed] [Google Scholar]
- Delbono O, 1992. Calcium current activation and charge movement in denervated mammalian skeletal muscle fibres. Journal of Physiology 451, 187–203. [PMC free article] [PubMed] [Google Scholar]
- Delbono O, 2003. Neural Control of Aging Skeletal Muscle. Aging Cell 2, 21–29. [PubMed] [Google Scholar]
- Delbono O, 2011a. Excitation-Contraction Coupling Regulation in Aging Skeletal Muscle, in: Lynch GS (Ed.), Sarcopenia-Age-Related Muscle Wasting and Weakness. Mechanisms and Treatment Springer, Victoria, pp. 113–134. [Google Scholar]
- Delbono O, 2011b. Expression and Regulation of Excitation-Contraction Coupling Proteins in Aging Skeletal Muscle. Curr Aging Sci 4(3), 248–59. [PMC free article] [PubMed] [Google Scholar]
- Delbono O, O’Rourke KS, Ettinger WH, 1995. Excitation-calcium release uncoupling in aged single human skeletal muscle fibers. Journal of Membrane Biology 148, 211–222. [PubMed] [Google Scholar]
- Delbono O, Renganathan M, Messi ML, 1997. Regulation of mouse skeletal muscle L-type Ca2+ channel by activation of the insulin-like growth factor-1 receptor. Journal of Neuroscience 17, 6918–6928. [PMC free article] [PubMed] [Google Scholar]
- Delbono O, Stefani E, 1993. Calcium current inactivation in denervated rat skeletal muscle fibres. Journal of Physiology 460, 173–183. [PMC free article] [PubMed] [Google Scholar]
- Distefano G, Goodpaster BH, 2018. Effects of Exercise and Aging on Skeletal Muscle. Cold Spring Harb Perspect Med 8(3):a029785. [PMC free article] [PubMed] [Google Scholar]
- Dobbins EG, Feldman JL, 1994. Brainstem network controlling descending drive to phrenic motoneurons in rat. J Comp Neurol 347, 64–86. [PubMed] [Google Scholar]
- Docherty JR, 2002. Age-related changes in adrenergic neuroeffector transmission. Autonomic Neuroscience 96, 8–12. [PubMed] [Google Scholar]
- Doherty TJ, Vandervoort AA, Taylor AW, Brown WF, 1993. Effects of motor unit losses on strength in older men and women. Journal of Applied Physiology 74, 868–874. [PubMed] [Google Scholar]
- Doxakis E, Howard L, Rohrer H, Davies AM, 2008. HAND transcription factors are required for neonatal sympathetic neuron survival. EMBO Rep 9, 1041–1047. [PMC free article] [PubMed] [Google Scholar]
- Du Y, Zhang M, Zhao W, Shu Y, Gao M, Zhuang Y, Yang T, Mu W, Li T, Li X, Sun F, Pan Z, Lu Y, 2017. Let-7a regulates expression of β1-adrenoceptors and forms a negative feedback circuit with the β1-adrenoceptor signaling pathway in chronic ischemic heart failure. Oncotarget 8, 8752–8764. [PMC free article] [PubMed] [Google Scholar]
- Dutta C, 1997. Significance of sarcopenia in the elderly. Journal of Nutrition 127, 992S–993S. [PubMed] [Google Scholar]
- Eftimie R, Brenner HR, Buonanno A, 1991. Myogenin and MyoD join a family of skeletal muscle genes regulated by electrical activity. Proc Natl Acad Sci U S A 88, 1349–1353. [PMC free article] [PubMed] [Google Scholar]
- Eichmann A, Brunet I, 2014. Arterial Innervation in Development and Disease. Science Translational Medicine 6, 252–252. [PubMed] [Google Scholar]
- Einsiedel LJ, Luff AR, 1992. Effect of partial denervation on motor units in the ageing rat medial gastrocnemius. Journal of the Neurological Sciences 112, 178–184. [PubMed] [Google Scholar]
- El-Salhy M, Sandström O, Holmlund F, 1999. Age-induced changes in the enteric nervous system in the mouse. Mechanisms of ageing and development 107, 93–103. [PubMed] [Google Scholar]
- Esler MD, Thompson JM, Kaye DM, Turner AG, Jennings GL, Cox HS, Lambert GW, Seals DR, 1995. Effects of aging on the responsiveness of the human cardiac sympathetic nerves to stressors. Circulation 91, 351–358. [PubMed] [Google Scholar]
- Faulkner JA, Brooks SV, Opiteck JA, 1993. Injury to Skeletal Muscle Fibers During Contractions: Conditions of Occurrence and Prevention. Physical Therapy 73, 911–921. [PubMed] [Google Scholar]
- Felten SY, Bellinger DL, Collier TJ, Coleman PD, Felten DL, 1987. Decreased sympathetic innervation of spleen in aged fischer 344 rats. Neurobiology of Aging 8, 159–165. [PubMed] [Google Scholar]
- Femminella GD, Rengo G, Komici K, Iacotucci P, Petraglia L, Pagano G, de Lucia C, Canonico V, Bonaduce D, Leosco D, Ferrara N, 2014. Autonomic dysfunction in Alzheimer’s disease: tools for assessment and review of the literature. J Alzheimers Dis 42, 369–377. [PubMed] [Google Scholar]
- Ferrucci L, Harris TB, Guralnik JM, Tracy RP, Corti MC, Cohen HJ, Penninx B, Pahor M, Wallace R, Havlik RJ, 1999. Serum IL-6 level and the development of disability in older persons. J Am Geriatr Soc 47, 639–646. [PubMed] [Google Scholar]
- Ferrucci L, Penninx BW, Volpato S, Harris TB, Bandeen-Roche K, Balfour J, Leveille SG, Fried LP, Md JM, 2002. Change in muscle strength explains accelerated decline of physical function in older women with high interleukin-6 serum levels. J Am Geriatr Soc 50, 1947–1954. [PubMed] [Google Scholar]
- Figueiredo VC, Markworth JF, Cameron-Smith D, 2017. Considerations on mTOR regulation at serine 2448: implications for muscle metabolism studies. Cell Mol Life Sci 74, 2537–2545. [PMC free article] [PubMed] [Google Scholar]
- Fish LA, Fallon JR, 2020. Multiple MuSK signaling pathways and the aging neuromuscular junction. Neuroscience Letters 731, 135014. [PMC free article] [PubMed] [Google Scholar]
- Frey D, Schneider C, Xu L, Borg J, Spooren W, Caroni P, 2000. Early and selective loss of neuromuscular synapse subtypes with low sprouting competence in motoneuron diseases. Journal of Neuroscience 20, 2534–2542. [PMC free article] [PubMed] [Google Scholar]
- Frontera WR, Suh D, Krivickas LS, Hughes VA, Goldstein R, Roubenoff R, 2000. Skeletal muscle fiber quality in older men and women. Am J Physiol Cell Physiol 279, C611–618. [PubMed] [Google Scholar]
- Fry CS, Lee JD, Mula J, Kirby TJ, Jackson JR, Liu F, Yang L, Mendias CL, Dupont-Versteegden EE, McCarthy JJ, Peterson CA, 2015. Inducible depletion of satellite cells in adult, sedentary mice impairs muscle regenerative capacity without affecting sarcopenia. Nat Med 21, 76–80. [PMC free article] [PubMed] [Google Scholar]
- Furlow JD, Watson ML, Waddell DS, Neff ES, Baehr LM, Ross AP, Bodine SC, 2013. Altered gene expression patterns in muscle ring finger 1 null mice during denervation- and dexamethasone-induced muscle atrophy. Physiol Genomics 45, 1168–1185. [PMC free article] [PubMed] [Google Scholar]
- Garcia-Prat L, Martinez-Vicente M, Perdiguero E, Ortet L, Rodriguez-Ubreva J, Rebollo E, Ruiz-Bonilla V, Gutarra S, Ballestar E, Serrano AL, Sandri M, Munoz- Canoves P, 2016. Autophagy maintains stemness by preventing senescence. Nature 529, 37–42. [PubMed] [Google Scholar]
- Geppetti P, De Rossi M, Mione M, Renzi D, Amenta F, 1988. Age-related changes in vasoactive intestinal polypeptide levels and distribution in the rat lung. Journal of neural transmission 74, 1–10. [PubMed] [Google Scholar]
- Goncalves DA, Silveira WA, Lira EC, Graca FA, Paula-Gomes S, Zanon NM, Kettelhut IC, Navegantes LC, 2012. Clenbuterol suppresses proteasomal and lysosomal proteolysis and atrophy-related genes in denervated rat soleus muscles independently of Akt. Am J Physiol Endocrinol Metab 302, E123–133. [PubMed] [Google Scholar]
- Gonzalez-Freire M, de Cabo R, Studenski S, Ferrucci L, 2014. The Neuromuscular Junction (NMJ): aging at the crossroad between nerves and muscle. Frontiers in Aging Neuroscience 6, 208. [PMC free article] [PubMed] [Google Scholar]
- Gonzalez A, Kirsch WG, Shirokova N, Pizarro G, Brum G, Pessah IN, Stern MD, Cheng H, Rios E, 2000a. Involvement of multiple intracellular release channels in calcium sparks of skeletal muscle. Proc Natl Acad Sci U S A 97, 4380–4385. [PMC free article] [PubMed] [Google Scholar]
- Gonzalez E, Messi ML, Delbono O, 2000b. The specific force of single intact extensor digitorum longus and soleus mouse muscle fibers declines with aging. J Membr Biol 178, 175–183. [PubMed] [Google Scholar]
- Gosztonyi G, Naschold U, Grozdanovic Z, Stoltenburg-Didinger G, Gossrau R, 2001. Expression of Leu-19 (CD56, N-CAM) and nitric oxide synthase (NOS) I in denervated and reinnervated human skeletal muscle. Microsc Res Tech 55, 187–197. [PubMed] [Google Scholar]
- Greensmith L, Vrbova G, 1996. Motoneurone survival: a functional approach. Trends Neurosci 19, 450–455. [PubMed] [Google Scholar]
- Griffin JW, Thompson WJ, 2008. Biology and pathology of nonmyelinating Schwann cells. Glia 56, 1518–1531. [PubMed] [Google Scholar]
- Gropp KE, 2017. Skeletal Muscle Toolbox. Toxicol Pathol 45, 939–942. [PubMed] [Google Scholar]
- Hadley D, Murphy T, Valladares O, Hannenhalli S, Ungar L, Kim J, Bucan M, 2006. Patterns of sequence conservation in presynaptic neural genes. Genome Biol 7, R105. [PMC free article] [PubMed] [Google Scholar]
- Hase A, Suzuki H, Arahata K, Akazawa C, 1999. Expression of human GFR alpha-1 (GDNF receptor) at the neuromuscular junction and myelinated nerves. Neurosci Lett 269, 55–57. [PubMed] [Google Scholar]
- Hashizume K, Kanda K, Burke R, 1988. Medial gastrocnemius motor nucleus in the rat: Age-related changes in the number and size of motoneurons. The Journal of Comparative Neurology 269, 425–430. [PubMed] [Google Scholar]
- Hendershot TJ, Liu H, Clouthier DE, Shepherd IT, Coppola E, Studer M, Firulli AB, Pittman DL, Howard MJ, 2008. Conditional deletion of Hand2 reveals critical functions in neurogenesis and cell type-specific gene expression for development of neural crest-derived noradrenergic sympathetic ganglion neurons. Developmental Biology 319, 179–191. [PMC free article] [PubMed] [Google Scholar]
- Henderson CE, Phillips HS, Pollock RA, Davies AM, Lemeulle C, Armanini M, Simmons L, Moffet B, Vandlen RA, Simpson L.C.c.t.S.L., Koliatsos VE, Rosenthal A, et al., 1994. GDNF: a potent survival factor for motoneurons present in peripheral nerve and muscle. Science 266, 1062–1064. [PubMed] [Google Scholar]
- Hendrickse P, Galinska M, Hodson-Tole E, Degens H, 2018. An evaluation of common markers of muscle denervation in denervated young-adult and old rat gastrocnemius muscle. Experimental Gerontology 106, 159–164. [PubMed] [Google Scholar]
- Hepple RT, 2018. When motor unit expansion in ageing muscle fails, atrophy ensues. J Physiol 596, 1545–1546. [PMC free article] [PubMed] [Google Scholar]
- Hirner S, Krohne C, Schuster A, Hoffmann S, Witt S, Erber R, Sticht C, Gasch A, Labeit S, Labeit D, 2008. MuRF1-dependent regulation of systemic carbohydrate metabolism as revealed from transgenic mouse studies. J Mol Biol 379, 666–677. [PubMed] [Google Scholar]
- Hoeldtke RD, Cilmi KM, 1985. Effects of aging on catecholamine metabolism. The Journal of Clinical Endocrinology & Metabolism 60, 479–484. [PubMed] [Google Scholar]
- Hook P, Li X, Sleep J, Hughes S, Larsson L, 1999. In vitro motility speed of slow myosin extracted from single soleus fibres from young and old rats. Journal of Physiology 520, 463–471. [PMC free article] [PubMed] [Google Scholar]
- Hwang AB, Brack AS, 2018. Chapter Ten - Muscle Stem Cells and Aging, in: Sassoon D (Ed.), Current Topics in Developmental Biology Academic Press, pp. 299–322. [PubMed] [Google Scholar]
- Ichimura Y, Kominami E, Tanaka K, Komatsu M, 2008. Selective turnover of p62/A170/SQSTM1 by autophagy. Autophagy 4, 1063–1066. [PubMed] [Google Scholar]
- Insel PA, 1993. Adrenergic receptors, G proteins, and cell regulation: implications for aging research. Exp Gerontol 28, 341–348. [PubMed] [Google Scholar]
- Jackson MJ, 2016. Reactive oxygen species in sarcopenia: Should we focus on excess oxidative damage or defective redox signalling? Mol Aspects Med 50:33–40. [PubMed] [Google Scholar]
- Jacobs BL, Abercrombie ED, Fornal CA, Levine ES, Morilak DA, Stafford IL, 1991. Single-unit and physiological analyses of brain norepinephrine function in behaving animals. Prog Brain Res 88, 159–165. [PubMed] [Google Scholar]
- Jang YC, Lustgarten MS, Liu Y, Muller FL, Bhattacharya A, Liang H, Salmon AB, Brooks SV, Larkin L, Hayworth CR, Richardson A, Van Remmen H, 2010. Increased superoxide in vivo accelerates age-associated muscle atrophy through mitochondrial dysfunction and neuromuscular junction degeneration. FASEB J 24, 1376–1390. [PMC free article] [PubMed] [Google Scholar]
- Janig W, 2012. Complex regional pain syndrome, 3rd ed. Academic, Amsterdam. [Google Scholar]
- Jensen-Daham C, Waldemar G, Jensen TS, Malmqvist L, Moeller MM, Andersen BB, Høgh P, Ballegaard M, 2015. Autonomic Dysfunction in Patients with Mild to Moderate Alzheimer’s Disease. Journal of Alzheimer’s Disease 47, 681–689. [PubMed] [Google Scholar]
- Jessen KR, Mirsky R, 2016. The repair Schwann cell and its function in regenerating nerves. The Journal of Physiology 594, 3521–3531. [PMC free article] [PubMed] [Google Scholar]
- Jimenez-Moreno R, Wang ZM, Guerring RC, Delbono O, 2008. Sarcoplasmic reticulum Ca2+ release declines in muscle fibers from aging mice. Biophys J 2008, 94(8):3178–88. [PMC free article] [PubMed] [Google Scholar]
- Johnson H, Mossberg K, Arvidsson U, Piehl F, Hokfelt T, Ulfhake B, 1995. Increase in alpha-CGRP and GAP-43 in aged motoneurons: A study of peptides, growth factors, and ChAT mRNA in the lumbar spinal cord of senescent rats with symptoms of hindlimb incapacities. The Journal of Comparative Neurology 359, 69–89. [PubMed] [Google Scholar]
- Jokl EJ, Blanco G, 2016. Disrupted autophagy undermines skeletal muscle adaptation and integrity. Mammalian Genome 27, 525–537. [PMC free article] [PubMed] [Google Scholar]
- Jones A, Teschendorff AE, Li Q, Hayward JD, Kannan A, Mould T, West J, Zikan M, Cibula D, Fiegl H, Lee S-H, Wik E, Hadwin R, Arora R, Lemech C, Turunen H, Pakarinen P, Jacobs IJ, Salvesen HB, Bagchi MK, Bagchi IC, Widschwendter M, 2013. Role of DNA Methylation and Epigenetic Silencing of HAND2 in Endometrial Cancer Development. PLoS Med 10, e1001551. [PMC free article] [PubMed] [Google Scholar]
- Kadhiresan VA, Hassett CA, Faulkner JA, 1996. Properties of single motor units in medial gastrocnemius muscles of adult and old rats. Journal of Physiology 493, 543–552. [PMC free article] [PubMed] [Google Scholar]
- Kallen RG, Sheng ZH, Yang J, Chen LQ, Rogart RB, Barchi RL, 1990. Primary structure and expression of a sodium channel characteristic of denervated and immature rat skeletal muscle. Neuron 4, 233–242. [PubMed] [Google Scholar]
- Kamwa V, Welch C, Hassan-Smith ZK, 2020. The endocrinology of sarcopenia and frailty. Minerva Endocrinol Online ahead of print. [PubMed] [Google Scholar]
- Kanda K, Hashizume K, 1989. Changes in properties of the medial gastrocnemius motor units in aging rats. Journal of Neurophysiology 1989, 737–746. [PubMed] [Google Scholar]
- Kanda K, Hashizume K, 1992. Factors causing difference in force output among motor units in the rat medial gastrocnemius muscle. Journal of Physiology 448, 677–695. [PMC free article] [PubMed] [Google Scholar]
- Kandinov B, Korczyn AD, Rabinowitz R, Nefussy B, Drory VE, 2011. Autonomic impairment in a transgenic mouse model of amyotrophic lateral sclerosis. Autonomic Neuroscience 159, 84–89. [PubMed] [Google Scholar]
- Kerman IA, Enquist LW, Watson SJ, Yates BJ, 2003. Brainstem substrates of sympatho-motor circuitry identified using trans-synaptic tracing with pseudorabies virus recombinants. J Neurosci 23, 4657–4666. [PMC free article] [PubMed] [Google Scholar]
- Khan MM, Lustrino D, Silveira WA, Wild F, Straka T, Issop Y, O’Connor E, Cox D, Reischl M, Marquardt T, Labeit D, Labeit S, Benoit E, Molgó J, Lochmüller H, Witzemann V, Kettelhut IC, Navegantes LCC, Pozzan T, Rudolf R, 2016. Sympathetic innervation controls homeostasis of neuromuscular junctions in health and disease. Proceedings of the National Academy of Sciences 113, 746–750. [PMC free article] [PubMed] [Google Scholar]
- Khuzakhmetova V, Bukharaeva E, 2020. Adrenaline Facilitates Synaptic Transmission by Synchronizing Release of Acetylcholine Quanta from Motor Nerve Endings. Cell Mol Neurobiol Online ahead of print. [PubMed] [Google Scholar]
- Koneczny I, Cossins J, Vincent A, 2014. The role of muscle-specific tyrosine kinase (MuSK) and mystery of MuSK myasthenia gravis. J Anat 224, 29–35. [PMC free article] [PubMed] [Google Scholar]
- Kuba K, 1970. Effects of catecholamines on the neuromuscular junction in the rat diaphragm. The Journal of Physiology 211, 551–570. [PMC free article] [PubMed] [Google Scholar]
- Landi F, Liperoti R, Russo A, Giovannini S, Tosato M, Capoluongo E, Bernabei R, Onder G, 2012. Sarcopenia as a risk factor for falls in elderly individuals: Results from the ilSIRENTE study. Clinical Nutrition 31, 652–658. [PubMed] [Google Scholar]
- Landi F, Marzetti E, Martone AM, Bernabei R, Onder G, 2014. Exercise as a remedy for sarcopenia. Current Opinion in Clinical Nutrition & Metabolic Care 17(1):25–31. [PubMed] [Google Scholar]
- Laplante M, Sabatini DM, 2012. mTOR signaling in growth control and disease. Cell 149, 274–293. [PMC free article] [PubMed] [Google Scholar]
- Larsson L, 1995. Motor units: remodeling in aged animals. Journals of Gerontology. Series A, Biological Sciences & Medical Sciences 50, 91–95. [PubMed] [Google Scholar]
- Larsson L, Ansved T, 1995. Effects of ageing on the motor unit. Progress in Neurobiology 45, 397–458. [PubMed] [Google Scholar]
- Larsson L, Ansved T, Edstrom L, Gorza L, Schiaffino S, 1991. Effects of age on physiological, immunohistochemical and biochemical properties of fast-twitch single motor units in the rat. Journal of Physiology 443, 257–275. [PMC free article] [PubMed] [Google Scholar]
- Larsson L, Degens H, Li M, Salviati L, Lee Y.i., Thompson W, Kirkland JL, Sandri M, 2019. Sarcopenia: Aging-Related Loss of Muscle Mass and Function. Physiological Reviews 99, 427–511. [PMC free article] [PubMed] [Google Scholar]
- Larsson L, Edstrom L, 1986. Effects of age on enzyme-histochemical fibre spectra and contractile properties of fast- and slow-twitch skeletal muscles in the rat. Journal of the Neurological Sciences 76, 69–89. [PubMed] [Google Scholar]
- Lau YS, Xu L, Gao Y, Han R, 2018. Automated muscle histopathology analysis using CellProfiler. Skelet Muscle 8(1), 32. [PMC free article] [PubMed] [Google Scholar]
- Le Panse B, Arlettaz A, Portier H, Lecoq AM, De Ceaurriz J, Collomp K, 2007. Effects of acute salbutamol intake during supramaximal exercise in women. Br J Sports Med 41, 430–434. [PMC free article] [PubMed] [Google Scholar]
- Leeuwenburgh C, Hansen P, Shaish A, Holloszy JO, Heinecke JW, 1998. Markers of protein oxidation by hydroxyl radical and reactive nitrogen species in tissues of aging rats. American Journal of Physiology 274, R453–461. [PubMed] [Google Scholar]
- Lefeuvre B, Crossin F, Fontaine-Perus J, Bandman E, Gardahaut MF, 1996. Innervation regulates myosin heavy chain isoform expression in developing skeletal muscle fibers. Mech Dev 58, 115–127. [PubMed] [Google Scholar]
- Lexell J, 1995. Human aging, muscle mass, and fiber type composition. Journals of Gerontology. Series A, Biological Sciences & Medical Sciences 50, 11–16. [PubMed] [Google Scholar]
- Li ST, Holmes C, Kopin IJ, Goldstein DS, 2003. Aging-related changes in cardiac sympathetic function in humans, assessed by 6–18F-fluorodopamine PET scanning. J Nucl Med 44, 1599–1603. [PubMed] [Google Scholar]
- Li W, Lam MT, Notani D, 2014. Enhancer RNAs. Cell Cycle 13, 3151–3152. [PMC free article] [PubMed] [Google Scholar]
- Li W, Notani D, Rosenfeld MG, 2016. Enhancers as non-coding RNA transcription units: recent insights and future perspectives. Nat Rev Genet 17, 207–223. [PubMed] [Google Scholar]
- Li Y, Thompson WJ, 2011. Nerve terminal growth remodels neuromuscular synapses in mice following regeneration of the postsynaptic muscle fiber. J Neurosci 31, 13191–13203. [PMC free article] [PubMed] [Google Scholar]
- Lindquist S, Stangel M, 2011. Update on treatment options for Lambert-Eaton myasthenic syndrome: focus on use of amifampridine. Neuropsychiatr Dis Treat 7, 341–349. [PMC free article] [PubMed] [Google Scholar]
- Lipsitz LA, Novak V, 2008. Aging and autonomic function, Third ed. Mayo Foundation, Rochester. [Google Scholar]
- Low PA, Bennarroch EE, 2008. Clinical Autonomic Disorders, 3rd ed. Lippincott Williams & Wilkins. [Google Scholar]
- Lynch GS, Ryall JG, 2008. Role of beta-adrenoceptor signaling in skeletal muscle: implications for muscle wasting and disease. Physiol Rev 88, 729–767. [PubMed] [Google Scholar]
- Lynch NA, Metter EJ, Lindle RS, Fozard JL, Tobin JD, Roy TA, Fleg JL, Hurley BF, 1999. Muscle quality. I. Age-associated differences between arm and leg muscle groups. Journal of Applied Physiology 86, 188–194. [PubMed] [Google Scholar]
- Machado J, Silveira WA, Gonçalves DA, Schavinski AZ, Khan MM, Zanon NM, Diaz MB, Rudolf R, Kettelhut IC, Navegantes LC, 2019. α−Calcitonin gene-related peptide inhibits autophagy and calpain systems and maintains the stability of neuromuscular junction in denervated muscles. Molecular Metabolism 28, 91–106. [PMC free article] [PubMed] [Google Scholar]
- Madisen L, Mao T, Koch H, Zhuo J. m., Berenyi A, Fujisawa S, Hsu Y-WA, Garcia Iii AJ, Gu X, Zanella S, Kidney J, Gu H, Mao Y, Hooks BM, Boyden ES, Buzsáki G, Ramirez JM, Jones AR, Svoboda K, Han X, Turner EE, Zeng H, 2012. A toolbox of Cre-dependent optogenetic transgenic mice for light-induced activation and silencing. Nat Neurosci 15(5):793–802. [PMC free article] [PubMed] [Google Scholar]
- Maeda K, Akagi J, 2016. Sarcopenia is an independent risk factor of dysphagia in hospitalized older people. Geriatrics & Gerontology International 16, 515–521. [PubMed] [Google Scholar]
- Maegawa S, Hinkal G, Kim HS, Shen L, Zhang L, Zhang J, Zhang N, Liang S, Donehower LA, Issa JP, 2010. Widespread and tissue specific age-related DNA methylation changes in mice. Genome Res 20, 332–340. [PMC free article] [PubMed] [Google Scholar]
- Masiero E, Agatea L, Mammucari C, Blaauw B, Loro E, Komatsu M, Metzger D, Reggiani C, Schiaffino S, Sandri M, 2009. Autophagy is required to maintain muscle mass. Cell Metab 10, 507–515. [PubMed] [Google Scholar]
- Mayeuf-Louchart A, Hardy D, Thorel Q, Roux P, Gueniot L, Briand D, Mazeraud A, Bougle A, Shorte SL, Staels B, Chretien F, Duez H, Danckaert A, 2018. MuscleJ: a high-content analysis method to study skeletal muscle with a new Fiji tool. Skelet Muscle 8(1), 25. [PMC free article] [PubMed] [Google Scholar]
- Mazzeo RS, Rajkumar C, Jennings G, Esler M, 1997. Norepinephrine spillover at rest and during submaximal exercise in young and old subjects. Journal of Applied Physiology 82, 1869–1874. [PubMed] [Google Scholar]
- Mei H, Ho MKC, Yung LY, Wu Z, Ip NY, Wong YH, 2011. Expression of Gαz in C2C12 cells restrains myogenic differentiation. Cellular Signalling 23, 389–397. [PubMed] [Google Scholar]
- Meitzen J, Perry AN, Westenbroek C, Hedges VL, Becker JB, Mermelstein PG, 2013. Enhanced Striatal β1-Adrenergic Receptor Expression Following Hormone Loss in Adulthood Is Programmed by Both Early Sexual Differentiation and Puberty: A Study of Humans and Rats. Endocrinology 154, 1820–1831. [PMC free article] [PubMed] [Google Scholar]
- Messéant J, Ezan J, Delers P, Glebov K, Marchiol C, Lager F, Renault G, Tissir F, Montcouquiol M, Sans N, Legay C, Strochlic L, 2017. Wnt proteins contribute to neuromuscular junction formation through distinct signaling pathways. Development 144, 1712–1724. [PubMed] [Google Scholar]
- Messi ML, Li T, Wang ZM, Marsh AP, Nicklas B, Delbono O, 2016. Resistance Training Enhances Skeletal Muscle Innervation Without Modifying the Number of Satellite Cells or their Myofiber Association in Obese Older Adults. J Gerontol A Biol Sci Med Sci 71, 1273–1280. [PMC free article] [PubMed] [Google Scholar]
- Miazaki M, Viana MP, Yang Z, Comin CH, Wang Y, da FCL, Xu X, 2015. Automated high-content morphological analysis of muscle fiber histology. Comput Biol Med 63, 28–35. [PMC free article] [PubMed] [Google Scholar]
- Minetti GC, Feige JN, Bombard F, Heier A, Morvan F, Nürnberg B, Leiss V, Birnbaumer L, Glass DJ, Fornaro M, 2014. Gαi2 Signaling Is Required for Skeletal Muscle Growth, Regeneration, and Satellite Cell Proliferation and Differentiation. Molecular and Cellular Biology 34, 619–630. [PMC free article] [PubMed] [Google Scholar]
- Mirotznik RR, Zheng X, Stanley EF, 2000. G-Protein Types Involved in Calcium Channel Inhibition at a Presynaptic Nerve Terminal. The Journal of Neuroscience 20, 7614–7621. [PMC free article] [PubMed] [Google Scholar]
- Mora A, Sandve GK, Gabrielsen OS, Eskeland R, 2016. In the loop: promoter-enhancer interactions and bioinformatics. Brief Bioinform 17, 980–995. [PMC free article] [PubMed] [Google Scholar]
- Moresi V, Williams AH, Meadows E, Flynn JM, Potthoff MJ, McAnally J, Shelton JM, Backs J, Klein WH, Richardson JA, Bassel-Duby R, Olson EN, 2010. Myogenin and Class II HDACs Control Neurogenic Muscle Atrophy by Inducing E3 Ubiquitin Ligases. Cell 143, 35–45. [PMC free article] [PubMed] [Google Scholar]
- Moriscot AS, Baptista IL, Bogomolovas J, Witt C, Hirner S, Granzier H, Labeit S, 2010. MuRF1 is a muscle fiber-type II associated factor and together with MuRF2 regulates type-II fiber trophicity and maintenance. Journal of Structural Biology 170, 344–353. [PMC free article] [PubMed] [Google Scholar]
- Moxham CM, Malbon CC, 1996. Insulin action impaired by deficiency of the G-protein subunit Gi[alpha]2. Nature 379, 840–844. [PubMed] [Google Scholar]
- Mu L, Sobotka S, Chen J, Su H, Sanders I, Adler CH, Shill HA, Caviness JN, Samanta JE, Beach TG, Arizona Parkinson’s Disease C, 2013. Alpha-synuclein pathology and axonal degeneration of the peripheral motor nerves innervating pharyngeal muscles in Parkinson disease. J Neuropathol Exp Neurol 72, 119–129. [PMC free article] [PubMed] [Google Scholar]
- Munver R, Volfson IA, 2006. Adrenal insufficiency: diagnosis and management. Curr Urol Rep 7, 80–85. [PubMed] [Google Scholar]
- Navegantes LC, Baviera AM, Kettelhut IC, 2009. The inhibitory role of sympathetic nervous system in the Ca2+-dependent proteolysis of skeletal muscle. Braz J Med Biol Res 42, 21–28. [PubMed] [Google Scholar]
- Ng AV, Callister R, Johnson DG, Seals DR, 1994. Sympathetic neural reactivity to stress does not increase with age in healthy humans. American Journal of Physiology-Heart and Circulatory Physiology 267, H344–H353. [PubMed] [Google Scholar]
- Nishiguchi S, Yamada M, Shirooka H, Nozaki Y, Fukutani N, Tashiro Y, Hirata H, Yamaguchi M, Tasaka S, Matsushita T, Matsubara K, Tsuboyama T, Aoyama T, 2016. Sarcopenia as a Risk Factor for Cognitive Deterioration in Community-Dwelling Older Adults: A 1-Year Prospective Study. Journal of the American Medical Directors Association 17, 372.e375–372.e378. [PubMed] [Google Scholar]
- O’Suilleabhain P, Low PA, Lennon VA, 1998. Autonomic dysfunction in the Lambert-Eaton myasthenic syndrome: serologic and clinical correlates. Neurology 50, 88–93. [PubMed] [Google Scholar]
- Ohara O, Gahara Y, Miyake T, Teraoka H, Kitamura T, 1993. Neurofilament deficiency in quail caused by nonsense mutation in neurofilament-L gene. J Cell Biol 121, 387–395. [PMC free article] [PubMed] [Google Scholar]
- Okada K, Inoue A, Okada M, Murata Y, Kakuta S, Jigami T, Kubo S, Shiraishi H, Eguchi K, Motomura M, Akiyama T, Iwakura Y, Higuchi O, Yamanashi Y, 2006. The muscle protein Dok-7 is essential for neuromuscular synaptogenesis. Science 312, 1802–1805. [PubMed] [Google Scholar]
- Okamoto LE, Biaggioni I, 2012. Chronic fatigue syndrome and the autonomic nervous system, in: Robertson D, Biaggioni I, Burnstock G, Low PA, Paton JFR (Ed.), Primer on the Autonomic Nervous System, 3rd ed. Academic, Amsterdam, pp. 531–534. [Google Scholar]
- Ooi W, Barrett S, Hossain M, Kelley-Gagnon M, Lipsitz LA, 1997. PAtterns of orthostatic blood pressure change and their clinical correlates in a frail, elderly population. JAMA 277, 1299–1304. [PubMed] [Google Scholar]
- Orimo S, Kanazawa T, Nakamura A, Uchihara T, Mori F, Kakita A, Wakabayashi K, Takahashi H, 2007. Degeneration of cardiac sympathetic nerve can occur in multiple system atrophy. Acta Neuropathol 113, 81–86. [PubMed] [Google Scholar]
- Owino W, Yang SY, Goldspink G, 2001. Age-related loss of skeletal muscle function and the inability to express the autocrine form of insulin-like growth factor-1 (MGF) in response to mechanical overload. FEBS Letters 505, 259–263. [PubMed] [Google Scholar]
- Palmer GJ, Ziegler MG, Lake CR, 1978. Response of norepinephrine and blood pressure to stress increases with age. Journal of Gerontology 33, 482–487. [PubMed] [Google Scholar]
- Pascualya M, Petrie EC, Brodkin K, Peskind ER, Veith RC, Raskind MA, 1999. Effects of advanced aging on plasma catecholamine responses to the cold pressor test. Neurobiology of Aging 20, 637–642. [PubMed] [Google Scholar]
- Payne AM, Delbono O, 2004. Neurogenesis of excitation-contraction uncoupling in aging skeletal muscle. Exerc Sport Sci Rev 32, 36–40. [PubMed] [Google Scholar]
- Payne AM, Messi ML, Zheng Z, Delbono O, 2006a. Motor neuron targeting of IGF-1 attenuates age-related external Ca2+-dependent skeletal muscle contraction in senescent mice. Journal of Physiology 570, 283–294. [PMC free article] [PubMed] [Google Scholar]
- Payne AM, Zheng Z, Messi ML, Milligan CE, Gonzalez E, Delbono O, 2006b. Motor neurone targeting of IGF-1 prevents specific force decline in ageing mouse muscle. J Physiol 570, 283–294. [PMC free article] [PubMed] [Google Scholar]
- Pellegrino MJ, Parrish DC, Zigmond RE, Habecker BA, 2011. Cytokines inhibit norepinephrine transporter expression by decreasing Hand2. Mol Cell Neurosci 46, 671–680. [PMC free article] [PubMed] [Google Scholar]
- Personius KE, Balice-Gordon RJ, 2000. Activity-dependent editing of neuromuscular synaptic connections. Brain Res Bull 53, 513–522. [PubMed] [Google Scholar]
- Pertl C, Eblenkamp M, Pertl A, Pfeifer S, Wintermantel E, Lochmuller H, Walter MC, Krause S, Thirion C, 2013. A new web-based method for automated analysis of muscle histology. BMC Musculoskelet Disord 14, 26. [PMC free article] [PubMed] [Google Scholar]
- Pette D, Staron RS, 2001. Transitions of muscle fiber phenotypic profiles. Histochem Cell Biol 115, 359–372. [PubMed] [Google Scholar]
- Pfleger J, Gresham K, Koch WJ, 2019. G protein-coupled receptor kinases as therapeutic targets in the heart. Nature Reviews Cardiology 16, 612–622. [PubMed] [Google Scholar]
- Phillips RJ, Powley TL, 2001. As the gut ages: Timetables for aging of innervation vary by organ in the Fischer 344 rat. Journal of Comparative Neurology 434, 358–377. [PubMed] [Google Scholar]
- Phillips RJ, Powley TL, 2007. Innervation of the gastrointestinal tract: patterns of aging. Autonomic Neuroscience 136, 1–19. [PMC free article] [PubMed] [Google Scholar]
- Picca A, Calvani R, Leeuwenburgh C, Coelho-Junior HJ, Bernabei R, Landi F, Marzetti E, 2019. Targeting mitochondrial quality control for treating sarcopenia: lessons from physical exercise. Expert Opin Ther Targets 23, 153–160. [PubMed] [Google Scholar]
- Pigna E, Renzini A, Greco E, Simonazzi E, Fulle S, Mancinelli R, Moresi V, Adamo S, 2018. HDAC4 preserves skeletal muscle structure following long-term denervation by mediating distinct cellular responses. Skeletal Muscle 8, 6. [PMC free article] [PubMed] [Google Scholar]
- Powley TL, 2008. Central Control of Autonomic Functions: Organization of the Autonomic Nervous System, in: Squire L, Berg D, Bloom F, du Lac S, Gosh A, Spitzer N (Eds.), Fundamental Neuroscience, Third ed. Elsevier Inc., Burlington, MA. USA, pp. 807–928. [Google Scholar]
- Purves-Smith FM, Sgarioto N, Hepple RT, 2014. Fiber typing in aging muscle. Exerc Sport Sci Rev 42, 45–52. [PubMed] [Google Scholar]
- Purves D, Augustine GJ, Fitzpatrick D, Hall WC, LaMantia AS, White LE, 2011. Neuroscience, 5th ed. Sinauer Associates, Inc., Massachusetts, USA. [Google Scholar]
- Radovanovic D, Peikert K, Lindström M, Domellöf FP, 2015. Sympathetic innervation of human muscle spindles. J Anat 226, 542–548. [PMC free article] [PubMed] [Google Scholar]
- Rauen T, Hedrich CM, Tenbrock K, Tsokos GC, 2013. cAMP responsive element modulator: a critical regulator of cytokine production. Trends in Molecular Medicine 19, 262–269. [PMC free article] [PubMed] [Google Scholar]
- Renganathan M, Sonntag WE, Delbono O, 1997. L-type Ca2+ channel-insulin-like growth factor-1 receptor signaling impairment in aging rat skeletal muscle. Biochemical & Biophysical Research Communications 235, 784–789. [PubMed] [Google Scholar]
- Rengo G, Pagano G, Vitale DF, Formisano R, Komici K, Petraglia L, Parisi V, Femminella GD, de Lucia C, Paolillo S, Cannavo A, Attena E, Pellegrino T, Dellegrottaglie S, Memmi A, Trimarco B, Cuocolo A, Filardi PP, Leosco D, Ferrara N, 2016. Impact of aging on cardiac sympathetic innervation measured by 123I-mIBG imaging in patients with systolic heart failure. European Journal of Nuclear Medicine and Molecular Imaging 43, 2392–2400. [PubMed] [Google Scholar]
- Révész D, Verhoeven JE, Milaneschi Y, de Geus EJCN, Wolkowitz OM, Penninx BWJH, 2014. Dysregulated physiological stress systems and accelerated cellular aging. Neurobiology of Aging 35, 1422–1430. [PubMed] [Google Scholar]
- Ricci A, Mariotta S, Greco S, Pallone G, Papale M, Bisetti A, 1997. Age-related changes of the noradrenergic innervation of rat tracheo-bronchial tree and pulmonary vasculature. Mechanisms of Ageing and Development 99, 245–255. [PubMed] [Google Scholar]
- Ristow M, 2014. Unraveling the Truth About Antioxidants: Mitohormesis explains ROS-induced health benefits. Nat Med 20, 709–711. [PubMed] [Google Scholar]
- Robertson D, Biaggioni I, Burnstock G, Low PA, Paton JFR, 2012. Primer on the Autonomic Nervous System, Third ed. Elsevier Inc., London, UK. [Google Scholar]
- Robinson TE, 1978. Electrical stimulation of the brain stem in freely moving rats: I. Effects on behavior. Physiol Behav 21, 223–231. [PubMed] [Google Scholar]
- Rodovalho GV, Drummond LR, Coimbra CC, 2020. Involvement of brainstem noradrenergic system in cutaneous heat loss during exercise. Brain Research Bulletin 164:372–379. [PubMed] [Google Scholar]
- Rodrigues ACZ, Messi ML, Wang Z-M, Abba MC, Pereyra A, Birbrair A, Zhang T, O’Meara M, Kwan P, Lopez EIS, Willis MS, Mintz A, Files DC, Furdui C, Oppenheim RW, Delbono O, 2018. The Sympathetic Nervous System Regulates Skeletal Muscle Motor Innervation and Acetylcholine Receptor Stability Acta Physiologica 225, e13195. [PMC free article] [PubMed] [Google Scholar]
- Rodrigues ACZ, Wang Z-M, Messi ML, Bonilla HJ, Liu L, Freeman WM, Delbono O, 2020. Heart and neural crest derivative 2-induced preservation of sympathetic neurons attenuates sarcopenia with aging. Journal of Cachexia, Sarcopenia and Muscle 11. [PMC free article] [PubMed] [Google Scholar]
- Rodrigues AZC, Wang Z-M, Messi ML, Delbono O, 2019. Sympathomimetics regulate neuromuscular junction transmission through TRPV1, P/Q- and N-type Ca2+ channels. Molecular and Cellular Neuroscience 95, 59–70. [PMC free article] [PubMed] [Google Scholar]
- Rohrer H, 2011. Transcriptional control of differentiation and neurogenesis in autonomic ganglia. Eur J Neurosci 34, 1563–1573. [PubMed] [Google Scholar]
- Rommel C, Bodine SC, Clarke BA, Rossman R, Nunez L, Stitt TN, Yancopoulos GD, Glass DJ, 2001. Mediation of IGF-1-induced skeletal myotube hypertrophy by PI(3)K/Akt/mTOR and PI(3)K/Akt/GSK3 pathways. Nat Cell Biol 3, 1009–1013. [PubMed] [Google Scholar]
- Rosenbaum DM, Rasmussen SG, Kobilka BK, 2009. The structure and function of G-protein-coupled receptors. Nature 459, 356–363. [PMC free article] [PubMed] [Google Scholar]
- Rosenberg, 1989. Summary comments. Am J Clin Nutr 50, 1231–1233. [Google Scholar]
- Rubinsztein DC, Marino G, Kroemer G, 2011. Autophagy and aging. Cell 146, 682–695. [PubMed] [Google Scholar]
- Rudolf R, Bogomolovas J, Strack S, Choi KR, Khan MM, Wagner A, Brohm K, Hanashima A, Gasch A, Labeit D, Labeit S, 2013. Regulation of nicotinic acetylcholine receptor turnover by MuRF1 connects muscle activity to endo/lysosomal and atrophy pathways. Age (Dordr) 35, 1663–1674. [PMC free article] [PubMed] [Google Scholar]
- Ruegg MA, Bixby JL, 1998. Agrin orchestrates synaptic differentiation at the vertebrate neuromuscular junction. Trends in Neurosciences 21, 22–27. [PubMed] [Google Scholar]
- Ryall JG, Lynch GS, 2008. The potential and the pitfalls of beta-adrenoceptor agonists for the management of skeletal muscle wasting. Pharmacol Ther 120, 219–232. [PubMed] [Google Scholar]
- Ryall JG, Schertzer JD, Alabakis TM, Gehrig SM, Plant DR, Lynch GS, 2008. Intramuscular {beta}2-agonist administration enhances early regeneration and functional repair in rat skeletal muscle after myotoxic injury. J Appl Physiol 105(1):165–72. [PubMed] [Google Scholar]
- Rychlik JL, Hsieh M, Eiden LE, Lewis EJ, 2005. Phox2 and dHAND transcription factors select shared and unique target genes in the noradrenergic cell type. J Mol Neurosci 27, 281–292. [PubMed] [Google Scholar]
- Ryu YK, Collins SE, Ho H-YH, Zhao H, Kuruvilla R, 2013. An autocrine Wnt5a-Ror signaling loop mediates sympathetic target innervation. Developmental Biology 377, 79–89. [PMC free article] [PubMed] [Google Scholar]
- Sakaguchi T, Okada M, Kitamura T, Kawasaki K, 1993. Reduced diameter and conduction velocity of myelinated fibers in the sciatic nerve of a neurofilament-deficient mutant quail. Neurosci Lett 153, 65–68. [PubMed] [Google Scholar]
- Sakellariou GK, Lightfoot AP, Earl KE, Stofanko M, McDonagh B, 2017. Redox homeostasis and age-related deficits in neuromuscular integrity and function. Journal of cachexia, sarcopenia and muscle 8, 881–906. [PMC free article] [PubMed] [Google Scholar]
- Sakuma K, Kinoshita M, Ito Y, Aizawa M, Aoi W, Yamaguchi A, 2016. p62/SQSTM1 but not LC3 is accumulated in sarcopenic muscle of mice. Journal of cachexia, sarcopenia and muscle 7, 204–212. [PMC free article] [PubMed] [Google Scholar]
- Salminen AL, Brandt A, Samuelsson K, Toytari O, Malmivaara A, 2009. Mobility devices to promote activity and participation: a systematic review. J Rehabil Med 41, 697–706. [PubMed] [Google Scholar]
- Salvatori S, Damiani E, Zorzato F, Volpe P, Pierobon S, Quaglino D, Salviati G, Margreth A, 1988. Denervation-induced proliferative changes of triads in rabbit skeletal muscle. Muscle & Nerve 11, 1246–1259. [PubMed] [Google Scholar]
- Santulli G, Iaccarino G, 2013. Pinpointing beta adrenergic receptor in ageing pathophysiology: victim or executioner? Evidence from crime scenes. Immunity & Ageing 10(1), 10. [PMC free article] [PubMed] [Google Scholar]
- Savitt JM, Jang SS, Mu W, Dawson VL, Dawson TM, 2005. Bcl-x Is Required for Proper Development of the Mouse Substantia Nigra. The Journal of Neuroscience 25, 6721–6728. [PMC free article] [PubMed] [Google Scholar]
- Schiaffino S, 2018. Muscle fiber type diversity revealed by anti-myosin heavy chain antibodies. The FEBS Journal 285, 3688–3694. [PubMed] [Google Scholar]
- Schiaffino S, Dyar KA, Ciciliot S, Blaauw B, Sandri M, 2013. Mechanisms regulating skeletal muscle growth and atrophy. FEBS J 280, 4294–4314. [PubMed] [Google Scholar]
- Schiaffino S, Reggiani C, 1994. Myosin isoforms in mammalian skeletal muscle. Journal of Applied Physiology 77, 493–501. [PubMed] [Google Scholar]
- Schiaffino S, Reggiani C, 1996. Molecular diversity of myofibrillar proteins: gene regulation and functional significance. Physiol Rev 76, 371–423. [PubMed] [Google Scholar]
- Schmidt M, Lin S, Pape M, Ernsberger U, Stanke M, Kobayashi K, Howard MJ, Rohrer H, 2009. The bHLH transcription factor Hand2 is essential for the maintenance of noradrenergic properties in differentiated sympathetic neurons. Dev Biol 329, 191–200. [PMC free article] [PubMed] [Google Scholar]
- Seibenhener ML, Babu JR, Geetha T, Wong HC, Krishna NR, Wooten MW, 2004. Sequestosome 1/p62 is a polyubiquitin chain binding protein involved in ubiquitin proteasome degradation. Mol Cell Biol 24, 8055–8068. [PMC free article] [PubMed] [Google Scholar]
- Sekulic A, Hudson CC, Homme JL, Yin P, Otterness DM, Karnitz LM, Abraham RT, 2000. A direct linkage between the phosphoinositide 3-kinase-AKT signaling pathway and the mammalian target of rapamycin in mitogen-stimulated and transformed cells. Cancer Res 60, 3504–3513. [PubMed] [Google Scholar]
- Silveira WA, Goncalves DA, Graca FA, Andrade-Lopes AL, Bergantin LB, Zanon NM, Godinho RO, Kettelhut IC, Navegantes LC, 2014. Activating cAMP/PKA signaling in skeletal muscle suppresses the ubiquitin-proteasome-dependent proteolysis: implications for sympathetic regulation. J Appl Physiol (1985) 117, 11–19. [PubMed] [Google Scholar]
- Smith LR, Barton ER, 2014. SMASH - semi-automatic muscle analysis using segmentation of histology: a MATLAB application. Skelet Muscle 4, 21. [PMC free article] [PubMed] [Google Scholar]
- Sonjak V, Jacob K, Morais JA, Rivera-Zengotita M, Spendiff S, Spake C, Taivassalo T, Chevalier S, Hepple RT, 2019. Fidelity of muscle fibre reinnervation modulates ageing muscle impact in elderly women. The Journal of Physiology, 597(19):5009–5023. [PubMed] [Google Scholar]
- Srikanthan P, Hevener AL, Karlamangla AS, 2010. Sarcopenia Exacerbates Obesity- Associated Insulin Resistance and Dysglycemia: Findings from the National Health and Nutrition Examination Survey III. PLOS ONE 5, e10805. [PMC free article] [PubMed] [Google Scholar]
- Stanzel S, Stubbusch J, Pataskar A, Howard MJ, Deller T, Ernsberger U, Tiwari VK, Rohrer H, Tsarovina K, 2016. Distinct roles of Hand2 in developing and adult autonomic neurons. Dev Neurobiol 76(10):1111–24. [PubMed] [Google Scholar]
- Straka T, Vita V, Prokshi K, Horner SJ, Khan MM, Pirazzini M, Williams MPI, Hafner M, Zaglia T, Rudolf R, 2018. Postnatal Development and Distribution of Sympathetic Innervation in Mouse Skeletal Muscle. Int J Mol Sci 19. [PMC free article] [PubMed] [Google Scholar]
- Straub V, Campbell KP, 1997. Muscular dystrophies and the dystrophin-glycoprotein complex. Curr Opin Neurol 10, 168–175. [PubMed] [Google Scholar]
- Studenski S, Perera S, Patel K, Rosano C, Faulkner K, Inzitari M, Brach J, Chandler J, Cawthon P, Connor EB, Nevitt M, Visser M, Kritchevsky S, Badinelli S, Harris T, Newman AB, Cauley J, Ferrucci L, Guralnik J, 2011. Gait speed and survival in older adults. JAMA 305, 50–58. [PMC free article] [PubMed] [Google Scholar]
- Tachi N, Ohya K, Chiba S, Nihira H, Minagawa K, 1995. Muscle involvement in congenital insensitivity to pain with anhidrosis. Pediatr Neurol 12, 264–266. [PubMed] [Google Scholar]
- Taetzsch T, Valdez G, 2018. NMJ maintenance and repair in aging. Current opinion in physiology 4, 57–64. [PMC free article] [PubMed] [Google Scholar]
- Tang H, Goldman D, 2006. Activity-dependent gene regulation in skeletal muscle is mediated by a histone deacetylase (HDAC)-Dach2-myogenin signal transduction cascade. Proc Natl Acad Sci U S A 103, 16977–16982. [PMC free article] [PubMed] [Google Scholar]
- Tang H, Shrager JB, Goldman D, 2019. Rapamycin protects aging muscle. Aging (Albany NY) 11, 5868–5870. [PMC free article] [PubMed] [Google Scholar]
- Tang S, Wong H-C, Wang ZM, Huang Y, Zou J, Zhuo Y, Pennati A, Gadda G, Delbono O, Yang JJ, 2011. Design and Application of a Class of Sensors to Monitor Ca2+ Dynamics in High Ca2+ Concentration Cellular Compartments. Proceedings of the National Academy of Sciences 2011 Sep 27. Epub 2011 Sep 13., 16265–16270. [PMC free article] [PubMed] [Google Scholar]
- Tchkonia T, Zhu Y, van Deursen J, Campisi J, Kirkland JL, 2013. Cellular senescence and the senescent secretory phenotype: therapeutic opportunities. The Journal of Clinical Investigation 123, 966–972. [PMC free article] [PubMed] [Google Scholar]
- Thoma A, Lightfoot AP, 2018. NF-kB and Inflammatory Cytokine Signalling: Role in Skeletal Muscle Atrophy. Adv Exp Med Biol 1088, 267–279. [PubMed] [Google Scholar]
- Tieland M, Trouwborst I, Clark BC, 2018. Skeletal muscle performance and ageing. J Cachexia Sarcopenia Muscle 9, 3–19. [PMC free article] [PubMed] [Google Scholar]
- Tournadre A, Vial G, Capel F, Soubrier M, Boirie Y, 2019. Sarcopenia. Joint Bone Spine 86, 309–314. [PubMed] [Google Scholar]
- Trimmer JS, Cooperman SS, Tomiko SA, Zhou JY, Crean SM, Boyle MB, Kallen RG, Sheng ZH, Barchi RL, Sigworth FJ, et al., 1989. Primary structure and functional expression of a mammalian skeletal muscle sodium channel. Neuron 3, 33–49. [PubMed] [Google Scholar]
- Tsarovina K, Reiff T, Stubbusch J, Kurek D, Grosveld FG, Parlato R, Schütz G, Rohrer H, 2010. The Gata3 Transcription Factor Is Required for the Survival of Embryonic and Adult Sympathetic Neurons. The Journal of Neuroscience 30, 10833–10843. [PMC free article] [PubMed] [Google Scholar]
- Tseng BS, Marsh DR, Hamilton MT, Booth FW, 1995. Strength and aerobic training attenuate muscle wasting and improve resistance to the development of disability with aging. J Gerontol A Biol Sci Med Sci 50 Spec No, 113–119. [PubMed] [Google Scholar]
- Tsentsevitsky AN, Kovyazina IV, Bukharaeva EA, 2019. Diverse Effects of Noradrenaline and Adrenaline on the Quantal Secretion of Acetylcholine at the Mouse Neuromuscular Junction. Neuroscience 423, 162–171. [PubMed] [Google Scholar]
- Upadhya B, Haykowsky MJ, Eggebeen J, Kitzman DW, 2015. Exercise intolerance in heart failure with preserved ejection fraction: more than a heart problem. J Geriatr Cardiol 12, 294–304. [PMC free article] [PubMed] [Google Scholar]
- Urasawa K, Murakami T, Yasuda H, 1991. Age-related alterations in adenylyl cyclase system of rat hearts. Jpn Circ J 55, 676–684. [PubMed] [Google Scholar]
- Urbancheck MG, Picken EB, Kalliainen LK, Kuzon WM, 2001. Specific force deficit in skeletal muscles of old rats is partially explained by the existence of denervated muscle fibers. Journal of Gerontology: Biological Sciences 56A, B191–B198. [PubMed] [Google Scholar]
- Vainshtein A, Tryon LD, Pauly M, Hood DA, 2015. Role of PGC-1alpha during acute exercise-induced autophagy and mitophagy in skeletal muscle. Am J Physiol Cell Physiol 308, C710–719. [PMC free article] [PubMed] [Google Scholar]
- Valdez G, Tapia JC, Kang H, Clemenson GD Jr., Gage FH, Lichtman JW, Sanes JR, 2010. Attenuation of age-related changes in mouse neuromuscular synapses by caloric restriction and exercise. Proc Natl Acad Sci U S A 107, 14863–14868. [PMC free article] [PubMed] [Google Scholar]
- Vasilaki A, Richardson A, Van Remmen H, Brooks SV, Larkin L, McArdle A, Jackson MJ, 2017. Role of nerve-muscle interactions and ROS in regulation of muscle proteostasis with aging. The Journal of Physiology 595, 6409–6415. [PMC free article] [PubMed] [Google Scholar]
- Veith RC, Featherstone JA, Linares OA, Halter JB, 1986. Age differences in plasma norepinephrine kinetics in humans. Journal of gerontology 41, 319–324. [PubMed] [Google Scholar]
- Vitale G, Cesari M, Mari D, 2016. Aging of the endocrine system and its potential impact on sarcopenia. Eur J Intern Med 35, 10–15. [PubMed] [Google Scholar]
- von Haehling S, Morley JE, Anker SD, 2010. An overview of sarcopenia: facts and numbers on prevalence and clinical impact. Journal of cachexia, sarcopenia and muscle 1, 129–133. [PMC free article] [PubMed] [Google Scholar]
- Wang Y, Hekimi S, 2015. Mitochondrial dysfunction and longevity in animals: Untangling the knot. Science 350, 1204–1207. [PubMed] [Google Scholar]
- Wang Z-M, Messi ML, Delbono O, 2000. L-type Ca2+ channel charge movement and intracellular Ca2+ in skeletal muscle fibers from aging mice. Biophysical Journal 78, 1947–1954. [PMC free article] [PubMed] [Google Scholar]
- Wang Z-M, Messi ML, Delbono O, 2002. Sustained overexpression of IGF-1 prevents age-dependent decrease in charge movement and intracellular calcium in mouse skeletal muscle. Biophysical Journal 82, 1338–1344. [PMC free article] [PubMed] [Google Scholar]
- Wang Z-M, Rodrigues ACZ, Messi ML, Delbono O, 2020a. Aging Blunts Sympathetic Neuron Regulation of Motoneurons Synaptic Vesicle Release Mediated by β1- and α2B-Adrenergic Receptors in Geriatric Mice. The Journals of Gerontology: Series A 75, 1473–1480. [PMC free article] [PubMed] [Google Scholar]
- Wang ZM, Messi ML, Grinevich V, Budygin E, Delbono O, 2020b. Postganglionic Sympathetic Neurons, but not Locus Coeruleus Optostimulation, Activates Neuromuscular Transmission in the Adult Mouse in Vivo. Mol Cell Neurosci, 109:103563. [PMC free article] [PubMed] [Google Scholar]
- Wang ZM, Zheng Z, Messi ML, Delbono O, 2005. Extension and magnitude of denervation in skeletal muscle from ageing mice. J Physiol 565, 757–764. [PMC free article] [PubMed] [Google Scholar]
- Weatherbee SD, Anderson KV, Niswander LA, 2006. LDL-receptor-related protein 4 is crucial for formation of the neuromuscular junction. Development 133, 4993–5000. [PubMed] [Google Scholar]
- Weindruch R, 1995. Interventions based on the possibility that oxidative stress contributes to sarcopenia. J Gerontol A Biol Sci Med Sci 50, 157–161. [PubMed] [Google Scholar]
- Wen Y, Murach KA, Vechetti IJ Jr., Fry CS, Vickery C, Peterson CA, McCarthy JJ, Campbell KS, 2018. MyoVision: software for automated high-content analysis of skeletal muscle immunohistochemistry. J Appl Physiol (1985) 124, 40–51. [PMC free article] [PubMed] [Google Scholar]
- White Z, White RB, McMahon C, Grounds MD, Shavlakadze T, 2016. High mTORC1 signaling is maintained, while protein degradation pathways are perturbed in old murine skeletal muscles in the fasted state. Int J Biochem Cell Biol 78, 10–21. [PubMed] [Google Scholar]
- Wokke JH, Jennekens FG, van den Oord CJ, Veldman H, Smit LM, Leppink GJ, 1990. Morphological changes in the human end plate with age. J Neurol Sci 95, 291–310. [PubMed] [Google Scholar]
- Xiang H-B, Liu C, Liu T-T, Xiong J, 2014. Central circuits regulating the sympathetic outflow to lumbar muscles in spinally transected mice by retrograde transsynaptic transport. International journal of clinical and experimental pathology 7, 2987–2997. [PMC free article] [PubMed] [Google Scholar]
- Xu Z, Feng X, Dong J, Wang Z-M, Lee J, Furdui C, Clark Files D, Beavers KM, Kritchevsky S, Milligan C, Jin J-P, Delbono O, Zhang T, 2017. Cardiac troponin T and fast skeletal muscle denervation in ageing. Journal of Cachexia, Sarcopenia and Muscle 8, 808–823. [PMC free article] [PubMed] [Google Scholar]
- Zhang C, Goto N, Suzuki M, Ke M, 1996. Age-related reductions in number and size of anterior horn cells at C6 level of the human spinal cord. Okajimas Folia Anatomica Japonica 73, 171–177. [PubMed] [Google Scholar]
- Zheng Z, Wang ZM, Delbono O, 2002. Insulin-like growth factor-1 increases skeletal muscle DHPR alpha 1S transcriptional activity by acting on the cAMP-response element-binding protein element of the promoter region. Journal of Biological Chemistry 277, 50535–50542. [PubMed] [Google Scholar]
- Zhu Y, Armstrong JL, Tchkonia T, Kirkland JL, 2014. Cellular senescence and the senescent secretory phenotype in age-related chronic diseases. Current Opinion in Clinical Nutrition & Metabolic Care 17, 324–328. [PubMed] [Google Scholar]